the Creative Commons Attribution 4.0 License.
the Creative Commons Attribution 4.0 License.
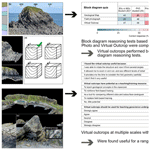
A role for virtual outcrop models in blended learning – improved 3D thinking and positive perceptions of learning
Adam J. Cawood
Virtual outcrop models are increasingly used in geoscience education to supplement field-based learning but their efficacy for teaching key 3D spatial thinking skills has been little tested. With the rapid increase in online digital learning resources and blended learning, most recently because of the global COVID-19 pandemic, understanding the role of virtual field environments in supporting and developing skills conventionally taught through field-based teaching has never been more critical. Here we show the efficacy of virtual outcrop models in improving 3D spatial thinking and provide evidence for positive perceptions amongst participants using virtual outcrops in teaching and learning. Our results show that, in a simple, multiple-choice scenario, participants were more likely to choose the 3D block diagram that best represents the structure when using a virtual outcrop (59 %) compared to more traditional representations, such as a geological map (50 %) or field photograph (40 %). We add depth to these results by capturing the perceptions of a cohort of students, within our full participant set, on the use of virtual outcrops for teaching and learning, after accessing a virtual field site and outcrops which they had previously visited during a day's field teaching. We also asked all participants if and how virtual outcrops could be used effectively for teaching and training, recording 87 % of positive responses. However, only 2 % of participants felt that virtual outcrops could potentially replace in-field teaching. We note that these positive findings signal significant potential for the effective use of virtual outcrops in a blended learning environment and for breaking barriers to increase the equality, diversity and inclusivity of geoscience field skills and teaching.
- Article
(6273 KB) - Full-text XML
- BibTeX
- EndNote
1.1 The importance of, and barriers to, field teaching for 3D spatial thinking
Field-based learning is known to enhance geological understanding (McKenzie, 1986; Elkins and Elkins, 2007; Tretinjak and Rigg, 2008) and is highly desired by many geoscience employers for its ability to enhance 3D spatial awareness and thinking (Butler, 2008). Field-based learning places rocks and concepts into a unique spatial context and provides a sense of the scale of geological processes. Through field-based mapping exercises, students make their own observations, generating working hypotheses in the form of 2D cross sections and geological maps that together test these hypotheses in 3D. This process develops 3D spatial reasoning skills, as students need to make interpretations and predictions based on what they see and measure in the field to explain the geology of a 3D volume.
There are, however, barriers to field access due to a range of logistical, financial and physical factors. Students with physical or other disabilities, for example, may find fieldwork difficult or impossible unless specific measures are put in place to enable access (e.g. Cooke et al., 1997; Gilley et al., 2015; Feig et al., 2019; Lang and Persico, 2019). Field-based training is expensive and, because students are increasingly expected to self-fund field trips at undergraduate level (Kent at al., 1997; Boyle et al., 2007; Giles et al., 2020), fieldwork may be prohibitively expensive for portions of the geoscience student population or may simply discourage study from the outset.
Assuming the logistical, financial and physical barriers to fieldwork can be circumvented, there are several other issues which may inhibit the effectiveness of field-based training. Students can find it difficult to keep up in group field activities when resources are limited, for example in back-of-the-class scenarios where students are only just arriving at the outcrop when the lecturer has finished the explanation, or weather conditions make it difficult to hear what is being said at the front, especially for those with hearing impairments. Students with little previous experience in the field or outdoors can be preoccupied and distracted by issues of safety, personal comfort, the physical demands of fieldwork and interactions with fellow students and instructors (Orion, 1993; Nairn et al., 2000; Boyle et al., 2007). A lack of in-course outdoor training, and acknowledgement of the uncertainties faced by such students in fieldwork participation, may also put such students off studying geoscience in the first place.
The recent Black in Geoscience movement, formed in the wake of Black Lives Matter, has also highlighted social and cultural issues around fieldwork participation, notably that many individuals from minority group communities lack experience in field and outdoor activities1. Further issues can include cultural norms in many western universities, such as a focus on socialising in bars and an evening drinking culture that may be exclusive on religious and other grounds (Rose, 1993; Miller, 2018; Guertin, 2019). Finally, safety concerns, unrecognised by many organisers, due to participants being black or brown in some areas and communities globally or being part of the LGBTQ+ community or being female may hinder or exclude participation and breach human rights (Giles et al., 2020). Such safety concerns outweigh the potential benefits of fieldwork. Finally, global public health concerns and safety in the light of the COVID-19 global pandemic add a further challenge to delivering field teaching. Although acknowledged as being an effective method for improving 3D thinking and spatial skills and a positive learning experience for many (Marques et al., 2003; Boyle et al., 2007), geological fieldwork may simply not be possible in the current situation, and for a number of individuals, it may potentially negatively impact their learning experience .
1.2 Classroom-based teaching of spatial skills and 3D thinking at university level
Field-based training works to develop 3D thinking and spatial reasoning skills, yet most teaching of geological skills, including spatial reasoning and 3D thinking, happens in the classroom. This is partly because of the issues discussed but mainly because of the practicalities of delivering undergraduate-level university courses. Classroom-based teaching also develops the skill of seeing information in 2D (e.g. maps and cross sections) or pseudo 3D (e.g. fence diagrams) and thinking in 3D. These 2D to 3D spatial skills underpin the concept of 3D thinking. The 2D representations of our 3D world are common, not only in the geoscience classroom (e.g. Newcombe and Shipley, 2015), but in everyday use, for example, maps for walking and guidebooks. Despite an increasingly digital and virtual world, 2D paper solutions are predominantly used in the classroom, and in other aspects of life, to convey 3D relationships, including those of geological geometries in the subsurface. Research has shown that students often have difficulty in visualising 3D geometries from these 2D materials (Ormand et al., 2014). These difficulties can be because of several factors, including a lack of training (e.g. how to read a map properly) and inherent difficulties with spatial thinking (e.g. Liben and Titus, 2012). Liben and Titus (2012) suggest that “Students arrive with underdeveloped spatial skills because there is inadequate attention to spatial thinking in both formal and informal education”. Yet spatial thinking has been identified as important for success in undergraduate STEM courses (Uttal and Cohen, 2012) and in geoscience (Riggs and Balliet, 2009; Titus and Horsman, 2009), but the evaluation of effective methods for teaching spatial skills and their development is limited to a few published investigations (Ormand et al., 2014; Gagnier et al., 2016; Ormand et al., 2017; Gold et al., 2018).
1.3 Virtual outcrops for supplementing field and classroom-based training
The rise in virtual worlds, including Google Earth and virtual gaming environments, and a parallel revolution in digital photogrammetric (structure from motion – SfM) techniques has changed 3D digital teaching possibilities. Virtual outcrops and hand samples can now be rapidly generated at low cost and presented through online teaching platforms. COVID-19 and the cancellation of many field courses globally has resulted in the rapid development of many virtual field trips, virtual outcrops and experiences in what had previously been an emergent area of expertise and teaching resources (e.g. Bond and Wightman, 2012; Whitmeyer, 2012; Granshaw and Duggan-Haas, 2012; Pringle, 2014; De Paor, 2016; Houghton et al., 2016; Carbonell Carrera et al., 2017a, b; Cawood and Bond, 2019). Many newly developed field trips and resources can be found online (e.g. NAGT website; https://nagt.org, last access: 9 April 2021). We do not review the efficacies of specific resources here but acknowledge that studies have shown that virtual field trips can improve learning outcomes and student experiences (e.g. Klippel et al., 2019) and gains in content knowledge (e.g. Markowitz et al., 2018; Mead et al., 2019). The blended learning environment in which online material, including virtual field trips (e.g. Whitmeyer and Dordevic, 2020), outcrops (e.g. Cawood and Bond, 2019) and thin sections (e.g. Herodotou et al., 2018) that support traditional classroom learning is here.
In this new era of blended learning with virtual fieldwork and online material, fully understanding the efficacies and limitations of these digital resources is increasingly important. The use of such digital resources is generally shown to have a positive impact on the student experience, and some studies have shown improved learning outcomes, but few studies have addressed the effectiveness of digital content for specifically teaching spatial awareness and 3D thinking in geoscience. In this study, we use web-based 3D virtual outcrop reconstructions and block diagrams of structures to test whether these virtual 3D models are more or less effective than traditional materials (photographs, geological maps and cross sections) for conveying 3D structural geology. We enhance our findings by asking several cohorts about their general perceptions of using the 3D virtual outcrop reconstructions, including in what contexts they found them useful and whether they felt that virtual outcrops should be included in curricula to improve learning, the learning environment and experience.
2.1 Questionnaires and online quizzes
An online set of quizzes and questionnaires was completed by two cohorts. Cohort 1 were final-year undergraduate geology students, in the same year and at the same university, and comprised 67 respondents who undertook an online, class-based exercise. No data on student background or expected degree grade was collected or considered in the analysis. Cohort 2 comprised individuals enrolled on the JISCMail GEOTECTONICS list server. This cohort is considered as being academic and industry structural and tectonic geology specialists representing a range of experience and career levels. Cohort 2 consisted of 156 respondents, and data on age and affiliation were collected to provide some context for analysis. The cohorts participated in three distinct elements that together formed the set of online questions and quizzes, with Cohort 1 completing all the elements and Cohort 2 completing elements 1 and 3. The first element (1) considered geospatial awareness, using three different representations of the same geological structure, namely a geological map, an oriented field photograph and a 3D virtual outcrop model. Participants looked at each representation and were asked to choose one of four block diagrams that they thought best reflected the structure observed. The second element (2) focused on a virtual field site and outcrops created by structure from motion (SfM) from a locality previously visited during a day field trip by Cohort 1. For this cohort, it allowed a direct comparison between their in-person visit to the site and their digital viewing of the field site and outcrops. The final element, undertaken by both cohorts, (3) focused on the perceptions of the participants in their use and experience of virtual outcrop models. The three elements are outlined in more detail below.
2.1.1 Geospatial awareness
In an online quiz, both cohorts were presented with a map, oriented field photograph and a 3D virtual outcrop model of the classic syncline structure in carbonates that forms a sea stack at Stackpole Quay, Pembrokeshire, SW Wales (see Cawood et al., 2017, for further context, and follow this link to the Virtual Outcrop model – https://www.e-rock.co.uk/stackpole, last access: 9 April 2021). Each representation appeared in the sequence of a map, field photograph and virtual outcrop model to participants, and associated with each representation were four block diagrams from which participants were required to choose the one which they thought was most representative of the syncline structure (Fig. 1). The virtual outcrop could be magnified and rotated; embedded into the virtual outcrop viewer were annotations denoting the orientation of the model (see https://www.e-rock.co.uk/stackpole, last access: 9 April 2021). The four block diagrams were similar in appearance, with only small differences in, for example, which limb of the syncline dips more steeply and the direction of plunge of the syncline (east or west).
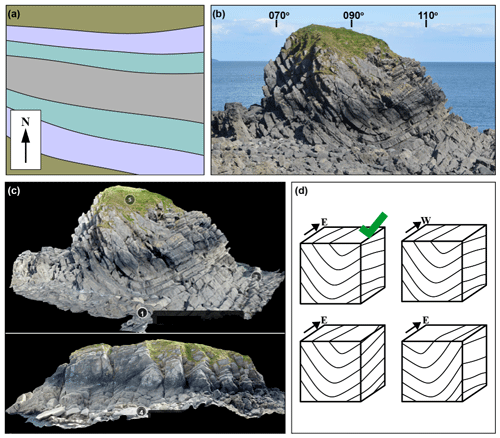
Figure 1Representations of the Stackpole syncline, Pembrokeshire. (a) Map view. (b) Field photograph with marked orientation directions. (c) Views of the virtual outcrop, with the top view pointed east and the bottom view pointed south. The numbered annotations seen on the virtual outcrop give the participants the directional information and are embedded into the viewer. (d) The four block diagrams from which participants had to choose the most accurate representation. The most accurate representation is the top left block diagram indicated with a green tick.
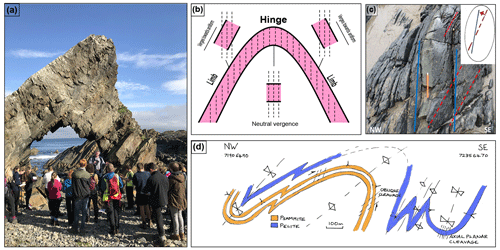
Figure 2Summary images of a day's field exercise to map folds in Dalradian metasediments and to use the concept of cleavage vergence to create a cross section. (a) Example student group being shown bedding–cleavage relationships in the field and how they can be used to predict an anticline of syncline along section. (b) Classroom-based course material showing cleavage–bedding relationships around a fold; the students employ this concept in the field to build a cross section. (c) Example from a field photograph of a bedding–cleavage relationship and interpretation and (d) worked solution cross section. Note that the course material and worked solutions were developed by Ian Alsop, Clare Bond and Rob Butler.
2.1.2 Post-field-trip virtual outcrop
This element, undertaken by the undergraduate cohort only (Cohort 1), built on an annual single day field trip for students in the third year of a 4-year UK undergraduate course. This day trip takes students to investigate Dalradian metasediment exposure along the Moray Coast, Scotland, near Macduff. The Dalradian metasediments are folded at a range of scales (from centimetres to hundreds of metres) in a series of upright folds. Sedimentary structures are retained in many units, and a combination of way-up criteria and cleavage development in pelitic layers allows the large-scale folding to be determined. Students are tasked, in the field, with building a cross section using bedding and cleavage orientations, together with the concept of vergence, to build up a picture of large-scale folding along the coastal section (Fig. 2). Students generally find the exercise challenging as the coastal exposure has sections that are missing or inaccessible and, therefore, requires visualisation skills and the confidence to make interpretations, correlating between isolated outcrops, to create a complete cross section. It is also the first time the students actively use the concept of vergence in the field, taking a theoretical concept presented as 2D images and cartoons in the classroom into a 3D physical space.
A total of 4 months after the day field trip, we provided students with a link to the virtual field site and outcrops (https://www.e-rock.co.uk/tarlair, last access: 9 April 2021). Embedded annotations, detailed virtual outcrops and photographs provide context and specific examples of cleavage–bedding relationships, particularly where model resolution does not allow these to be observed directly (Fig. 3). The students were given computer laboratory time and access to complete the online exercise, but participation was voluntary, and it was emphasised to the students that the work would be completed anonymously and would not be formally or informally assessed in the context of their degree study. We asked the participants to respond to eight online statements (Fig. 5a) related to their perceptions of the virtual outcrop model and how it impacted their understanding of structures at the site. The students responded to these statements by selecting their response from the following choices: strongly agree, agree, neutral, disagree and strongly disagree.
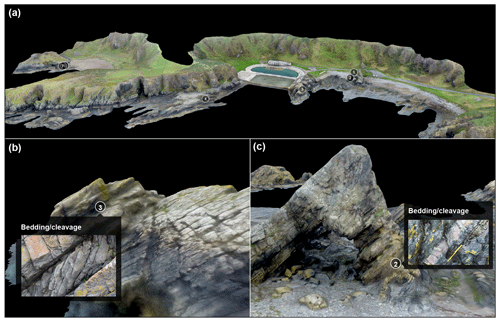
Figure 3Virtual field site and outcrops. The panels show (a) the whole field site, and numbered annotations magnify the sites in greater detail, with a higher-resolution field photograph pop-up for the student, as shown in (b), and in panel (c), the annotation labelled 2 links to a detailed virtual outcrop model of the bedding–cleavage relationship.
2.1.3 General perceptions
In the final element, participants in Cohort 2 answered two questions about how the virtual outcrops had either enhanced or not enhanced their understanding of the fold geometry and, in the former case, to which visualisation they attributed this understanding, i.e. the virtual outcrop, oriented field photograph and geological map combination, geological map or oriented field photograph. Then participants in both cohorts answered three questions about how they perceived the use of virtual outcrops models generally and in terms of their use for teaching and research. Multiple answers could be chosen from a range of specified options.
Note that, for Cohort 1, each exercise was completed sequentially on campus in a computer laboratory space through an online quiz and with access to the virtual outcrops and field sites. For Cohort 2 elements 1 and 3 were completed sequentially through a single online quiz.
3.1 Geospatial awareness and choice of block diagrams
The geospatial awareness element was completed by both cohorts, making it possible to amalgamate and compare the results across a range of career stages and affiliations. We divided the results into the following career stage and/or affiliation categories: (1) undergraduate and Masters students (82 participants), doctoral students (26), professionals (28), academics (70) and other respondents (7). The response data in Fig. 4 is broken down into affiliation categories, and these effectively show the difference between experience levels, i.e. there is a general increase in correct response rate with increasing experience. When combining data from Cohort 1 and 2, the participants who are students in a bachelor or Masters of science degree programme (e.g. Cohort 1 and those that identified themselves in this way in Cohort 2) perform worse than other categories in the identification of the most representative block diagram, whilst the self-identified professionals in Cohort 2 do the best. Overall, participants found identifying the correct block diagram from the field photograph the most difficult, with only a 40 % success rate. Participants were better able to identify the most representative block diagram to match the virtual outcrop model and map. Overall, participants performed best when using the virtual outcrop model (59 %) compared to the map (50 %; Fig. 4). We also note that, in contrast to the overall results, both doctoral students and academics were more likely, 65 % and 74 % respectively, to choose the correct block diagram after viewing the geological map.
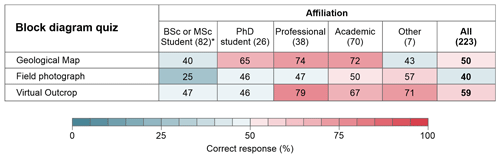
Figure 4Responses to the geospatial awareness test in which participants had to identify the most representative block diagram when given a (1) geological map, (2) field photograph or (3) virtual outcrop model. Data from both cohorts are represented in the figure. The amalgamated data are split into five affiliation categories, and the actual numbers of participants in each affiliation category are given in parentheses after each category. Percentages are given for the correct response.
3.2 Post-fieldwork virtual outcrop access perceptions
Cohort 1 generally responded positively to the eight statements about use of the virtual outcrop model to enhance their learning and understanding (Fig. 5a). For six of the statements, the majority of the participants, between 60 %–83 %, answered that they strongly agree or agree. These statements focused on the visualisation of geometries, for example; the participants felt that the virtual outcrop model improved their understanding of structural geometries, with the majority of students (60 %) selecting either strongly agree or agree from the response choices (Fig. 5a). The two statements that were not answered so positively were, firstly, “has changed my ideas about the structural geometries at Macduff since visiting the area last year”, to which the most chosen category was neutral (40 %), with an approximately symmetrical split of answers around this majority neutral response. The other statement that did not elicit a positive response was: “allows me to differentiate between psammite and pelite more easily than in the field” (31 % responses were for both the neutral and disagree options). We investigate the potential reasons for these responses in the discussion (Sect. 4.4).
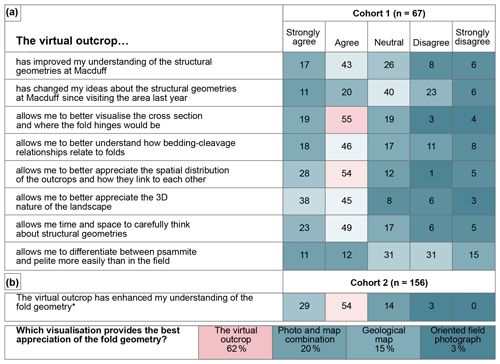
Figure 5Responses by participants to questions on the value of virtual outcrops to enhance learning and understanding. (a) Responses by Cohort 1 to eight statements given in column 1. (b) Responses by Cohort 2 to a single question on the enhancement of understanding and which of the representations gives the best visualisation of the fold geometry. Responses are given as percentages to the possible answers; see Fig. 4 for the colour scale.
3.3 General perceptions of virtual outcrop models
Having viewed the virtual outcrop model of the Stackpole syncline, Cohort 2, similar to Cohort 1, felt that the virtual outcrop enhanced their understanding of the fold geometry, with 83 % of participants agreeing (54 %) or strongly agreeing (29 %) with the statement (Fig. 5a). The majority of the Cohort 2 participants also felt that the virtual outcrop provided the best appreciation of the fold geometry (62 %) in comparison to the field photograph and geological map combined (20 %), the geological map (15 %) or the oriented field photograph (3 %; Fig. 5a).
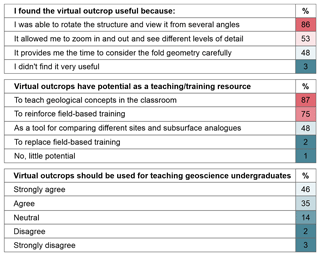
Figure 6Responses, summarised as percentages, from both cohorts to question on virtual outcrop use and value for teaching.
Both cohorts (1 and 2) answered questions about why they found the virtual outcrop useful, if they felt virtual outcrops could be used as a teaching and training resource and, finally, if they felt they should be used. Figure 6 shows the responses to these questions as a percentage of participants in the whole cohort. The participants found the virtual outcrop useful mainly because they could rotate and view the structure from several angles (86 %), with a further 53 % of respondents indicating that they found the ability to zoom in and out to see different levels of detail useful. Only 3 % of all 223 participants did not find the virtual outcrop useful. Cohort 1 also agreed or strongly agreed (72 %) with the statement that “the virtual outcrop allows me time and space to carefully think about structural geometries”. A total of 87 % of participants felt that virtual outcrops had potential to teach geological concepts in the classroom, with 75 % of participant agreeing that they could be used to reinforce field-based training. In contrast, only 1 % of participants felt that virtual outcrops had no potential as a teaching or training resource. However, only 2 % felt that virtual outcrops had the potential to replace field-based teaching.
In our experiment, we found that a virtual outcrop model aided participants' ability to identify the block diagram that best represented the cropping out structure from a choice of four. In conjunction with these results, we found that the participants perceived significant value in the use of virtual outcrops as a teaching and training resource. Here we discuss the limitations of our spatial thinking experiment, the challenges in soliciting opinions and perceptions, the implications of our results for spatial thinking and learning, the perceptions of participants and reasons behind these, with a view to inform spatial thinking and learning, and, finally, to suggest how virtual outcrops might be used in a blended teaching environment for the benefit of all but particularly to improve equality, diversity and inclusivity in geoscience field work.
4.1 Experimental limitations
As is common to all experiments, our experimental methods likely impacted our findings to some degree, which we discuss here. The experiment was delivered online, and the software we used for this online delivery did not allow randomisation of questions. Hence, the sequential representations of the Stackpole syncline structure as a map, field photograph and virtual outcrop in the spatial thinking element were always presented to participants in the same order. This is likely to have resulted in some growing knowledge through the sequential exposure to these different representations. In order to help mitigate this issue, we changed the location of the correct block diagram, in the set of four, so that participants had to critically investigate elements of the representation they were viewing to match them against the details in the different block diagrams. Future studies that both engage a greater number of participants and that include randomisation of question order and visual representations would be useful to support or challenge the findings presented here on spatial thinking using virtual outcrops.
4.2 Challenges in soliciting and interpreting perceptions of participants
Spatial thinking experiments that show learning are hard to construct because of a series of confounding issues (Ormand et al., 2017), and like several other studies (e.g. Gold et al., 2018), we focus on the perceptions of participants to add value and depth to our results. In our interpretation of the perceptions of participants, we acknowledge that the perception of the value of learning for an individual may not correspond to an actual impact on their learning, as noted by Oloruntegbe and Alam (2010) and DiPiro (2010). However, we also note that perception of value and positive experiences aid learning (López-Pérez et al., 2011; Gillen et al., 2011). In this context, we interpret the perceptions of participants purely as positive, negative or neutral experiences, without deriving any implication for affective improvements in learning or geological understanding, which provides an indication of how to create positive learning experiences and environments.
Given the nature of the study and the form of questions in the experiment, participants may have felt that ,in being asked about their perceptions of virtual outcrop use, the study was hypothesising or expecting positive responses. This may also be viewed as being reinforced by some of the statements provided. As far as possible, we attempted to mitigate these confounding elements by the free and anonymous participation of individuals and the opportunity to choose negative statements. In this respect, we believe that we provided participants with free choice to respond negatively, neutrally or positively to how they found virtual outcrops and their potential use for teaching and training. Indeed, some participants, albeit a small number, did choose negative responses.
4.3 Spatial thinking
The results of the spatial thinking element of the exercise showed that participants performed better when using the virtual outcrop model (59 %) compared to the other representations. Although overall the virtual outcrop model proved to be the representation that resulted in the most correct block diagram choice responses, both doctoral students and academics were more likely to choose the correct block diagram after viewing the geological map. We suggest that this may be due doctoral students and academics being familiar with map-based problems rather than due to working in a digital 3D environment, which may be more familiar to undergraduate and Masters students through exposure while gaming and for industry professionals working in digital 3D interpretation software. These suggestions are suppositions, and further research is needed from which to draw robust conclusions. Irrespective of the variance in response between affiliation categories, it is increasingly common for geoscientists to spend a significant proportion of their time at a computer, and visualising geology digitally in 3D is common practice in the industry. Virtual outcrops provide a clear opportunity to bridge the gap between 2D representations of 3D information, common in classrooms, and the interpretation and analysis of geoscience data and information within a digital 3D environment in industrial applications.
The ability to work between 2D representations and 3D is identified as a specific and important skill for geoscientists (Ormand et al., 2017). Even in advanced professional geoscience software, for example, for seismic interpretation, the user is often working between 2D and 3D space because of the constraints of desktop digital environments for fully immersive 3D interpretation and interaction. Building skills to manipulate and interrogate virtual outcrops and going between 2D representations and views into 3D space in a digital environment, in the context of future working environments, would appear to be a good thing. It will build digital skills analogous to those used in software for subsurface data sets. The ability to think in 3D, as highlighted by employers as a key skill for geoscientists (e.g. Butler, 2008), is likely to be enhanced by working in 3D space (Woods et al., 2016; Carbonell et al., 2017). Given the need to work between 2D and 3D representations, the development of virtual environments, in which not only the map view is possible but section views can also be seen, would be likely to aid such skill development. These types of section views are available in digital block model generators, such as pynoddy (e.g. Wellmann et al., 2016), and the ability to move between maps and sections and draped realisations of these (e.g. Bond and Wightman, 2012) and other data, for example, synthetic seismic imagery (e.g. Anell et al., 2016) in a virtual field environment, would be likely to add learning value.
4.4 Perceptions of virtual outcrop value for 3D spatial thinking and learning
Positive responses to statements were made by both cohorts about virtual outcrop use. These included being able to rotate and view the structure from different angles (86 %) and being able to zoom in and out to see different levels of detail (53 %). Only 3 % of the 223 participants across both cohorts responded that they did not find the virtual outcrop useful.
With the students that formed Cohort 1, we were able to investigate their perceptions of access to virtual outcrops and a virtual field site model after visiting the site on a day field trip. Their responses to the virtual field environment were positive, particularly for elements that involved 3D spatial understanding, with the majority of participants strongly agreeing or agreeing to statements such as: “allows me to better appreciate the 3D nature of the landscape” (83 %), “allows me to better visualise the cross-section and where the fold hinges would be”(74 %), and “allows me to better appreciate the spatial distribution of the outcrops and how they link to each other” (82 %). Cohort 1, undergraduate students, perceived that access to the virtual outcrop and field site helped their 3D appreciation for the field site and structures.
Of the statements, two that Cohort 1 responded to focused less on 3D spatial understanding aspects and rather on an overarching understanding and rock identification; responses to these questions were less positive. The two statements that were responded to less positively were, firstly, “has changed my ideas about the structural geometries at Macduff since visiting the area last year”, to which the most chosen category was neutral (40 %), with an approximately symmetrical split of answers around this majority neutral response. We interpret this as the virtual outcrop confirming existing opinions from the participants' field experience rather than informing any radical reinterpretation. This response was expected, given the virtual outcrop use in a context where students have previously developed a conceptual model in the field.
The other statement that did not elicit a positive response was “allows me to differentiate between psammite and pelite more easily than in the field”, with 46 % of participants disagreeing or strongly disagreeing with this statement; we believe this highlights two things. First, the importance of observing rocks and their textures in the field, acknowledging that photography and virtual outcrop models are not the same as viewing something in its physical reality. The ease of transition between scales in the field may also be critical to effective learning and assimilation of information. In the case of the virtual field site used here, a higher level of rock texture detail in the photogrammetric reconstruction may have improved the ability of participants to distinguish between rock types in the virtual outcrop. Image quality issues have also been highlighted in other studies (Rogers, 2020). Although, we note that, when surveyed after the fieldwork, students cite difficulties in understanding bedding–cleavage relationships (42 %) and internally visualising the cross section (41 %) as the most challenging aspects of the Macduff field exercise.
Second, for geological field observations, a range in scale is often important. Virtual outcrops with tiled photographs allow observations at a range of scales. The resolution of the unoccupied aerial vehicle (UAV) photography and UAV and SfM technology is improving at a significant rate, making higher-resolution models at different scales increasingly possible, even with limited viewing hardware. The balance in the near term will be in rendering models with a high enough resolution to be effective, while understanding the limitations of internet/cloud access to virtual outcrop resources and the participants' own operating hardware. In terms of the range of possible scales, virtual outcrops have the potential to provide a transition across multiple scales, from satellite imagery to the hand lens view. With the potential assimilation of thin section imagery and beyond into electron microscopy in an integrated environment, they could surpass in-field possibilities in terms of the range of observational scales available. Web-based viewers with seamless transitions between views and across a range of scales at this level is not yet available, however.
In the field, participants are fully embedded in the 3D space. The scale of the landscape relative to the viewer is easily apparent. With the use of a map, participants can take this in-landscape viewpoint into a bird's eye view if they have accomplished 3D thinking and spatial skills. It is difficult for even the best virtual outcrops and virtual field sites to compete with the experience of being physically present in a landscape and having the associated appreciation of relative scale. In terms of viewpoints, the participant in the field is limited by local topography in their ability to gain different viewpoints and perspectives. In a virtual outcrop or virtual field site effectively any viewpoint is possible, and the translation from within the model to a bird's eye (map) view is straightforward. Participants from both cohorts recognised being able to rotate and view the structure from different angles (86 %) as a key benefit of virtual outcrop models. Virtual reality environments also increasingly allow participants to be embedded in the scene; this, we believe, will become a significant area of development in virtual geoscience field trip environments. In summary, carefully built virtual environments have a lot to offer in terms of the range of scales of observation and viewpoints and, in some areas, offer more than the field experience. The value of these elements in terms of 3D spatial thinking is perceived positively by participants in our study who used virtual outcrops.
4.5 A role for virtual outcrop models in blended learning
Perceptions across all participants on the potential for virtual outcrop use as a teaching or training resource was high, with 87 % of participants agreeing with the statement that virtual outcrops have potential to teach geological concepts in the classroom and to reinforce field teaching 75 %. These positive responses to statements were backed up with a further 81 % of all participants strongly agreeing or agreeing to the statement that “Virtual outcrops should be used for teaching geoscience undergraduates”. Only 5 % of all participants disagreed or strongly disagreed with this statement, and only 1 % indicated that virtual outcrops have little to no potential as a teaching or training resource. The potential role of virtual outcrop models for teaching and positive perceptions of virtual outcrop use for teaching and learning across a range (age and career level) of geoscientists is clearly documented in our study.
Advantages and disadvantages outlined in our discussion on perceptions of the value of virtual outcrops for 3D spatial thinking and learning (Sect. 4.4) suggest that virtual outcrops and field experiences are not a replacement for fieldwork but can significantly enhance learning and can be used as part of a positive learning experience. Survey responses support this, with only 2 % of participants agreeing with the statement that virtual outcrop models have the potential to replace field-based training. The positive responses to how virtual outcrops may enhance learning and training opportunities do, however, call for them to have a place alongside field training in a blended learning environment. The educational benefits identified in this study and the positive perceptions of learning experiences and benefits support this argument. But there are clearly other advantages in terms of equality, diversity and inclusivity in geoscience, as raised in the introduction, where virtual outcrop models and virtual field sites have several major advantages over in-field experience, as follows: (1) providing virtual access for those who cannot go to the field because of disability, financial constraints, etc. or who find it difficult to operate effectively in the field; (2) providing access to global locations and viewpoints to areas that are simply not accessible during traditional fieldwork for anyone; and (3) providing the ability to work at your own pace, with less pressure, fewer distractions or concerns. To improve, equality, diversity and inclusion in geoscience and field access, virtual outcrops models and virtual field sites, we believe, have an indisputable role.
Blended learning, flipped learning and other models that integrate self-learning practices with taught elements are growing with increasing access to education at a range of ages and career stages. For example, the University of the Third Age and Massive Online Open Courses (MOOCS). These have become possible in part through the growing digital revolution and an associated ease of accessibility. For geoscience and for geological field work in particular, digital delivery has, until COVID-19, been a slowly evolving field which has experienced significant acceleration in learning and practice in 2020. We argue that, in a post-COVID-19 world, virtual outcrops and field experiences have a key role to play in blended learning environments to improve 3D spatial thinking and learning, in creating positive learning experiences and to significantly improve equality, diversity and inclusivity in geoscience field work.
Access to the digital outcrop models created by Clare Bond and Adam Cawood are available via Stackpole Syncline, Pembrokeshire, SW Wales, at https://www.e-rock.co.uk/stackpole (eRock, 2021a) and were acquired and processed by Adam Cawood.
Moreover, the Tarlair coastal exposures, Moray, NE Scotland, are available at https://www.e-rock.co.uk/tarlair (eRock, 2021b) and were acquired and processed by Adam Cawood and Clare Bond.
CEB conceived the experiment in collaboration with AJC; together, they determined the questions and designed the online questionnaire. AJC built the online questionnaire and created an initial analysis of the results through creation of the main figures and tables in the paper. Together, the authors discussed the results and narrative, which AJC outlined based around the study results. CEB then wrote the paper, which AJC edited and commented on.
The authors declare that they have no conflict of interest.
This article is part of the special issue “Virtual geoscience education resources”. It is not associated with a conference.
The authors would like to thank all undergraduate participants in Cohort 1 for participating in the exercise and providing their perceptions and thoughts. Similarly, we thank those members of the GEO-TECTONICS JISCMail server who responded to the online questionnaire; without these responses and participants' views, this paper would not have been possible.
This paper was edited by Steven Rogers and reviewed by Chris King and Edward McGowan.
Anell, I., Lecomte, I., Braathen, A., and Buckley, S. J.: Synthetic seismic illumination of small-scale growth faults, paralic deposits and low-angle clinoforms: A case study of the Triassic successions on Edgeøya, NW Barents Shelf, Mar. Petrol. Geol., 77, 625–639, https://doi.org/10.1016/j.marpetgeo.2016.07.005, 2016.
Bond, C. and Wightman, R.: Beyond map view: teaching the conceptualisation and visualisation of geology through 3D and 4D geological models, Planet, 25, 7–15, https://doi.org/10.11120/plan.2012.00250007, 2012.
Boyle, A., Maguire, S., Martin, A., Milsom, C., Nash, R., Rawlinson, S., Turner, A., Wurthmann, S., and Conchie, S.: Fieldwork is good: The student perception and the affective domain, J. Geogr. Higher Educ., 31, 299–317, https://doi.org/10.1080/03098260601063628, 2007.
Butler, R.: Teaching geoscience through fieldwork, Higher Education Academy Subject Centre for Geography, Earth and Environmental Sciences, ISBN 1-84102-1458-8, available at: https://s3.eu-west-2.amazonaws.com/assets.creode.advancehe-document-manager/documents/hea/private/gees_guides_rb_teaching_geoscience_1568036903.pdf (last access: 9 April 2021), 2008.
Carbonell Carrera, C. and Bermejo Asensio, L. A.: Augmented reality as a digital teaching environment to develop spatial thinking, Cartogr. Geogr. Inf. Sc., 44, 259–270, https://doi.org/10.1080/15230406.2016.1145556, 2017.
Carbonell Carrera, C., Avarvarei, B. V., Chelariu, E. L., Draghia, L., and Avarvarei, S. C.: Map-reading skill development with 3D technologies, J. Geogr., 116, 197–205, https://doi.org/10.1080/00221341.2016.1248857, 2017.
Cawood, A. J. and Bond, C. E.: eRock: An Open-Access Repository of Virtual Outcrops for Geoscience Education, GSA Today, 29, 36–37, https://doi.org/10.1130/GSATG373GW.1, 2019.
Cawood, A. J., Bond, C. E., Howell, J. A., Butler, R. W., and Totake, Y.: LiDAR, UAV or compass-clinometer? Accuracy, coverage and the effects on structural models, J. Struct. Geol., 98, 67–82, https://doi.org/10.1016/j.jsg.2017.04.004, 2017.
Cooke, M. L., Anderson, K. S., and Forrest, S. E.: Creating accessible introductory geology field trips, J. Geosci. Educ., 45, 4–9, https://doi.org/10.5408/1089-9995-45.1.4, 1997.
De Paor, D. G.: Virtual rocks, GSA Today, 26, 4–11, https://doi.org/10.1130/GSATG257A.1, 2016.
DiPiro, J. T.: Student learning: perception versus reality, Am. J. Pharm. Educ., 74, 63, https://doi.org/10.5688/aj740463, 2010.
Elkins, J. T. and Elkins, N. M.: Teaching geology in the field: Significant geoscience concept gains in entirely field-based introductory geology courses, J. Geosci.-Czech, 55, 126–132, https://doi.org/10.5408/1089-9995-55.2.126, 2007.
eRock: Stackpole Syncline, available at: https://www.e-rock.co.uk/stackpole, last access: 14 April 2021a.
eRock: Tarlair coastal exposure, available at: https://www.e-rock.co.uk/tarlair, last access: 14 April 2021b.
Feig, A. D., Atchison, C., Stokes, A., and Gilley, B.: Achieving inclusive field-based education: Results and recommendations from an accessible geoscience field trip, Journal of the Scholarship of Teaching and Learning, 19, 66–87, https://doi.org/10.14434/josotl.v19i1.23455, 2019.
Gagnier, K. M., Shipley, T. F., Tikoff, B., Garnier, B. C., Ormand, C., Atit, K., and Resnick, I.: Training Spatial Skills in Geosciences: A Review of Tests and Tools, in: 3D Structural Interpretation: Earth, Mind, and Machine, edited by: Krantz, B., Ormand, C., and Freeman, B., American Association of Petroleum Geologists, Tulsa, OK, United States, 111, 7–23, https://doi.org/10.1306/13561983M1113668, 2016.
Giles, S., Jackson, C., and Stephen, N.: Barriers to fieldwork in undergraduate geoscience degrees, Nature Reviews Earth and Environment, 1, 77–78, https://doi.org/10.1038/s43017-020-0022-5, 2020.
Gillen, A., Wright, A., and Spink, L.: Student perceptions of a positive climate for learning: a case study, Educational Psychology in Practice, 27, 65–82, https://doi.org/10.1080/02667363.2011.549355, 2011.
Gilley, B., Atchison, C., Feig, A., and Stokes, A.: Impact of inclusive field trips, Nat. Geosci., 8, 579–580, https://doi.org/10.1038/ngeo2500, 2015.
Gold, A. U., Pendergast, P. M., Ormand, C. J., Budd, D. A., and Mueller, K. J.: Improving spatial thinking skills among undergraduate geology students through short online training exercises, Int. J. Sci. Educ., 40, 2205–2225, https://doi.org/10.1080/09500693.2018.1525621, 2018.
Granshaw, F. D. and Duggan-Haas, D.: Virtual fieldwork in geoscience teacher education: Issues, techniques, and models, in: Google Earth and Virtual Visualizations in Geoscience Education and Research, edited by: Whitmeyer, S. J., Bailey, J. E., De Paor, D. G., and Ornduff, T., The Geological Society of America, Boulder, CO 80301-9140, 492, 285–303, https://doi.org/10.1130/2012.2492(20), 2012.
Guertin, L.: Want to be more inclusive? Stop making geology conferences about the beer, AGU GeoEd Trek blog, available at: https://blogs.agu.org/geoedtrek/2019/12/08/inclusive-agu-conferences/ (last access: 9 April 2021), 2019.
Herodotou, C., Muirhead, D. K., Aristeidou, M., Hole, M. J., Kelley, S., Scanlon, E., and Duffy, M.: Blended and online learning: a comparative study of virtual microscopy in Higher Education, Interact. Learn. Envir., 28, 713–728, https://doi.org/10.1080/10494820.2018.1552874, 2018.
Houghton, J., Gordon, C., Craven, B., Robinson, A., Lloyd, G. E., and Morgan, D. J.: How to Make a Virtual Landscape with Outcrops for Use in Geoscience Teaching, AGUFM, ED51H-0847, available at: https://ui.adsabs.harvard.edu/abs/2016AGUFMED51H0847H/abstract (last access: 9 April 2021), 2016.
Kent, M., Gilbertsone, D., and Hunt, C.: Fieldwork in geography teaching: a critical review of the literature and approaches, J. Geogr. Higher Educ., 21, 313–332, https://doi.org/10.1080/03098269708725439, 1997.
Klippel, A., Zhao, J., Oprean, D., Wallgrün, J. O., Stubbs, C., La Femina, P., and Jackson, K. L.: The value of being there: Toward a science of immersive virtual field trips, Virtual Real.-London, 24, 753–770, https://doi.org/10.1007/s10055-019-00418-5, 2019.
Lang, N. P. and Persico, L. P.: Challenges and approaches for creating inclusive field courses for students with an autism spectrum disorder, J. Geosci.-Czech, 67, 345–350, https://doi.org/10.1080/10899995.2019.1625996, 2019.
Liben, L. S. and Titus, S. J.: The importance of spatial thinking for geoscience education: Insights from the crossroads of geoscience and cognitive science, in: Earth and Mind II: A Synthesis of Research on Thinking and Learning in the Geosciences, edited by: Kastens, K. A. and Manduca, C. A., The Geological Society of America, Boulder, CO 80301-9140, 486, 51–70, https://doi.org/10.1130/2012.2486(10), 2012.
López-Pérez, M. V., Pérez-López, M. C., and Rodríguez-Ariza, L.: Blended learning in higher education: Students' perceptions and their relation to outcomes, Comput. Educ., 56, 818–826, https://doi.org/10.1016/j.compedu.2010.10.023, 2011.
Markowitz, D. M., Laha, R., Perone, B. P., Pea, R. D., and Bailenson, J. N.: Immersive virtual reality field trips facilitate learning about climate change, Front. Psychol., 9, 2364, https://doi.org/10.3389/fpsyg.2018.02364, 2018.
Marques, L., Praia, J. O., and Kempa, R.: A study of students' perceptions of the organisation and effectiveness of fieldwork in earth sciences education, Res. Sci. Technol. Educ., 21, 265–278, https://doi.org/10.1080/0263514032000127275, 2003.
McKenzie, G. D.: The Importance of Field Trips, Journal of College Science Teaching, 16, 17–20, 1986.
Mead, C., Buxner, S., Bruce, G., Taylor, W., Semken, S., and Anbar, A. D.: Immersive, interactive virtual field trips promote science learning, J. Geosci.-Czech, 67, 131–142, https://doi.org/10.1080/10899995.2019.1565285, 2019.
Miller, A.: In some disciplines, heavy drinking is part of the culture, That can be a problem, Science Magazine blog, American Association for the Advancement of Science, 1200 New York Ave, NW, Washington, DC, 20005, https://doi.org/10.1126/science.caredit.aaw3020, 2018.
Nairn, K., Higgitt, D., and Vanneste, D.: International perspectives on fieldcourses, J. Geogr. Higher Educ., 24, 246–254, https://doi.org/10.1080/713677382, 2000.
Newcombe, N. S. and Shipley, T. F.: Thinking About Spatial Thinking: New Typology, New Assessments, in: Studying Visual and Spatial Reasoning for Design Creativity, edited by: Geor, J., Springer, Dordrecht, The Netherlands, 179–192, https://doi.org/10.1007/978-94-017-9297-4_10, 2015.
Oloruntegbe, K. O. and Alam, G. M.: Evaluation of 3d environments and virtual realities in science teaching and learning: The need to go beyond perception referents, Sci. Res. Essays, 5, 948–954, 2010.
Orion, N. A.: A model for the development and implementation of field trips as an integral part of the science curriculum, School Science and Mathematics, 93, 325–331, available at: https://eric.ed.gov/?id=EJ480158 (last access: 9 April 2021), 1993.
Ormand, C. J., Manduca, C., Shipley, T. F., Tikoff, B., Harwood, C. L., Atit, K., and Boone, A. P.: Evaluating geoscience students' spatial thinking skills in a multi-institutional classroom study, J. Geosci.-Czech, 62, 146–154, https://doi.org/10.5408/13-027.1, 2014.
Ormand, C. J., Shipley, T. F., Tikoff, B., Dutrow, B., Goodwin, L. B., Hickson, T., Atit, K., Gagnier, K., and Resnick, I.: The Spatial Thinking Workbook: A research-validated spatial skills curriculum for geology majors, J. Geosci.-Czech, 65, 423–434, https://doi.org/10.5408/16-210.1, 2017.
Pringle, J. K.: Educational egaming: the future for geoscience virtual learners? Geology Today, 30, 147–150, https://doi.org/10.1111/gto.12058, 2014.
Riggs, E. M. and Balliet, R. N.: A role for mental rotations in field-based problem solving, The Geological Society of America, Boulder, CO 80301-9140, 41, 195 pp., 2009.
Rogers, S. L.: Cheap, accessible, and virtual experiences as tools for immersive study: a proof of concept study, Research in Learning Technology, 28, 2416, https://doi.org/10.25304/rlt.v28.2416, 2020.
Rose, G.: Feminism and geography: the limits of geographical knowledge, Polity Press, Cambridge, UK, 1993.
Titus, S. J. and Horsman, E.: Characterizing and improving spatial visualization skills, J. Geosci.-Czech, 57, 242–254, https://doi.org/10.5408/1.3559671, 2009.
Tretinjak, C. A. and Riggs, E. M.: Enhancement of geology content knowledge through field-based instruction for pre-service elementary teachers, J. Geosci.-Czech, 56, 422–433, https://doi.org/10.5408/jge_nov2008_riggs_422, 2008.
Uttal, D. H. and Cohen, C. A.: Spatial thinking and STEM education: When, why and how?, in: Psychology of learning and motivation, edited by: Ross, B., Academic Press, Oxford, UK, 57, 147–181, https://doi.org/10.1016/b978-0-12-394293-7.00004-2, 2012.
Wellmann, J. F., Thiele, S. T., Lindsay, M. D., and Jessell, M. W.: pynoddy 1.0: an experimental platform for automated 3-D kinematic and potential field modelling, Geosci. Model Dev., 9, 1019–1035, https://doi.org/10.5194/gmd-9-1019-2016, 2016.
Woods, T. L., Reed, S., Hsi, S., Woods, J. A., and Woods, M. R.: Pilot study using the augmented reality sandbox to teach topographic maps and surficial processes in introductory geology labs, J. Geosci.-Czech, 64, 199–214, https://doi.org/10.5408/15-135.1, 2016.
Whitmeyer, S. J.: Google Earth and Virtual Visualizations in Geoscience Education and Research, The Geological Society of America, Boulder, CO 80301-9140, USA, https://doi.org/10.1130/9780813724928, 468 pp., 2012.
Whitmeyer, S. J. and Dordevic, M.: Creating virtual geologic mapping exercises in a changing world, Geosphere, 17, 226–243, https://doi.org/10.1130/GES02308.1, 2020.
Although we do not wish to stereotype, and recognise that many minority group individuals have experience, we also recognise that outdoor pursuits and field geology have traditionally been dominated by white males.