the Creative Commons Attribution 4.0 License.
the Creative Commons Attribution 4.0 License.
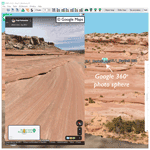
Virtual field trips utilizing virtual outcrop: construction, delivery and implications for the future
Jessica H. Pugsley
John A. Howell
Adrian Hartley
Simon J. Buckley
Rachel Brackenridge
Nicholas Schofield
Gail Maxwell
Magda Chmielewska
Kari Ringdal
Nicole Naumann
Joris Vanbiervliet
The advent of photorealistic, 3D computer models of cliff sections (virtual outcrops) has improved the immersive nature of virtual geological field trips. As the COVID-19 pandemic led to widespread national and international travel restrictions, virtual field trips (VFTs) became practical and essential substitutes for traditional field trips and accelerated the development of VFTs based on virtual outcrop data. This contribution explores two such VFTs delivered to a masters level Integrated Petroleum Geoscience course at the University of Aberdeen. These VFTs are based on traditional field trips that are normally run to the Spanish Pyrenees and Utah (USA). The paper summarizes the delivery mechanism for VFTs based on virtual outcrops and examines student perception, gauged primarily through questionnaires and learning outcomes. The VFTs were run in LIME, a software specifically designed for the interpretation of 3D models and the delivery of VFTs. Overall, the student perception was very positive and comparable to satisfaction with the conventional trips. Staff feedback and student assessments suggest that the learning outcomes were satisfied and highlight the value of this method of teaching for students who are unable to attend the field trip and as an addition for those who can.
- Article
(9764 KB) - Full-text XML
-
Supplement
(882 KB) - BibTeX
- EndNote
Field trips are a fundamental component of most geoscience degrees. Prior to COVID-19, in the UK for a geology degree to be accredited by the Geological Society of London there was a requirement for 60 d of fieldwork. Similar requirements exist in other countries. Significant emphasis is placed on the skills that are acquired through time spent in the field, observing rocks and structures in their natural habitat. In recent years there has been increasing recognition that, for a variety of reasons, fieldwork is not equally accessible to all students (Giles et al., 2020), and there have been increased efforts to provide digital alternatives, termed virtual field trips (VFTs).
The term VFT has a broad range of implications and interpretations. VFTs can range from a slide show via a Google Earth tour of localities to a full immersive experience using 3D virtual outcrop models. The form of immersive technology can vary from desktop to virtual reality (VR) headsets (e.g. Klippel et al., 2020) with augmented reality also emerging (e.g. Gazcón et al., 2018). Virtual field trips can also be subdivided into “location based” or “thematic” trips (Fig. 1a). Location based trips are the most comparable to a traditional field trips and are focused on the geology of a specific geographic area. Thematic or geographically unconfined VFTs follow a specific topic and visit outcrop examples from several distinct locations. These are more similar to traditional classroom taught course but are augmented with outcrop examples from across the world.
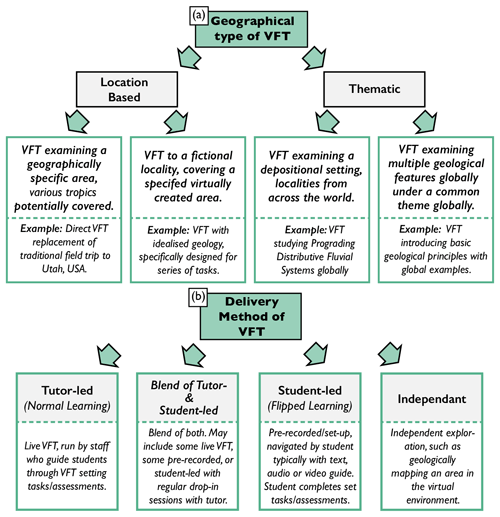
Figure 1Outline of types and delivery methods of VFTs. (a) Types of VFT separated between location-based trips to a specific locality and thematic trips spanning global localities with a common theme. Examples are given. (b) Delivery method of VFT divided into tutor-led VFTs and student-led VFTs as well as the blended spectrum between. Examples are given.
Virtual field trips can be further subdivided based upon the degree of tutor involvement at the time of delivery (Fig. 1b). There is a spectrum of trip types, which range from real-time tutor-led trips through to releasing students into an immersive space and allowing them to explore for themselves, in their own time. The different trip types may be more suited to specific topics, particular learning outcomes and the level of student experience.
A virtual outcrop (VO), sometimes called digital outcrop model or virtual outcrop model, is a photorealistic model of a geological outcrop. Virtual outcrops first appeared in the late 1990s (Xu et al., 2000) and became more popular with the advent of lidar (light detection and ranging) (Bellian et al., 2005; Pringle et al., 2006; Buckley et al., 2008, 2013). Over the last 8 years there has been a proliferation of virtual outcrops due to the dual emergence of remote piloted vehicles (RPVs, commonly termed drones) and structure from motion (SfM) photogrammetry (Buckley et al., 2017; Harrald et al., 2021; Howell et al., 2021). Together, these two technologies have made virtual outcrops widely available across the geosciences.
Virtual outcrops have traditionally been used for research purposes (e.g. Enge et al., 2010; Rittersbacher et al., 2015, and many others). In recent years, virtual outcrops have started to be used in virtual field trips (VFTs) (e.g. Argles et al., 2015; Tibaldi et al., 2020; Bond and Cawood, 2021; Gregory et al., 2022), although their acceptance has yet to become widespread, and they are typically used to provide supplementary material. Even during the COVID-19 pandemic, the VFTs run by many groups did not contain VOs, instead adopting conventional teaching methods (slides, PowerPoint, etc.) or Google Earth and/or geographic information system (GIS) tools (e.g. Whitmeyer and Dordevic, 2021; Bosch, 2021; Barth et al., 2022).
To date, there has been little systematic evaluation comparing virtual-outcrop-based VFTs and real-world field trips with similar conditions, primarily because of the logistical challenges of running parallel trips under controlled experimental setups. The onset of the COVID-19 global pandemic and associated lockdowns from March 2020 forced the implementation of VFTs on a far broader scale and created the opportunity for such studies. Within this contribution, we examine the outcomes of two VFTs that were run as replacements for traditional field courses on the Integrated Petroleum Geoscience (IPG) MSc classes of 2020 and 2021. The VFTs ran in real time over 11 d (Utah) and 5 d (Pyrenees). They were based on well-established traditional trips to Utah and the Spanish Pyrenees. The Utah course has run for over 25 years, and the Pyrenees trip has run in various forms since 2010. The VFTs were built on an extensive set of VOs and other data collected by the authors for research purposes over the last 15 years (e.g. Eide et al., 2015; Howell et al., 2014; Phillips et al., 2021). The Utah VFT was run twice (September 2020 and August 2021), and the Pyrenees VFT was run once in October 2020.
The aim of this contribution is to summarize the learning from these VFTs to help ensure that future VFTs are more effective at achieving the similar learning outcomes to traditional field trip. The specific objectives are the following: (1) to present the workflow for building and running VFTs, developed over the past 5 years; (2) to assess the effectiveness of VFTs through student interaction; and (3) to review student perception of VFT and how this compares to the traditional field trips.
The concept of teaching geological field skills in a virtual environment is not new (Hurst, 1998; Stainfield et al., 2000); however, over the past decade VFTs have become increasingly popular, reaching a high during the COVID-19 pandemic. The advantages and disadvantages of traditional VFTs are well established, with numerous studies discussing the benefits and challenges of their delivery and reception. However, the developments in virtual outcrops, as well as associated platforms (e.g., LIME; Buckley et al., 2019) and cloud-hosted web viewers (e.g. V3Geo, Buckley et al., 2022), and the advent of immersive reality and VR headsets illustrate that this field is advancing rapidly.
2.1 Advantages of VFTs
VFTs enable a greater volume of data to be presented at different scales from the small (e.g. scanning electron microscope (SEM) images, thin sections and hand samples) to the large (e.g. virtual outcrops, DEMs and maps) (Hurst, 1998; Arrowsmith et al., 2005; Atchison and Feig, 2011; Çaliskan, 2011; Bailey et al., 2012). This range of scale is linked to enhancements in 3D understanding (Hurst, 1998; Bond and Cawood, 2021), which is a key skill within geoscience. VFTs also have the capacity to be geographically independent (based on a common theme) and permit a higher number of individuals to attend (Stainfield et al., 2000; Dolphin et al., 2019). They are weather and tide independent (Dolphin et al., 2019), resulting in them being logistically easier to plan, deliver and timetable (Hurst, 1998; Butler, 2008), as well as being associated with lower carbon emissions (Schott, 2017). Largely owing to the absence of long distance travel, VFTs are also typically more time efficient than their conventional counterparts (Ramasundaram et al., 2005). VFTs also allow participants to easily revisit localities to cement learning (Hurst, 1998).
Inclusivity is a key advantage of VFTs. They reduce the financial burden associated with travel (Stainfield et al., 2000; Fletcher et al., 2002; Ramasundaram et al., 2005; Jacobson et al., 2009; Litherland and Stott, 2012; Dolphin et al., 2019) and offer participation to individuals with restricted physical access (Stainfield et al., 2000; Atchison and Feig, 2011; Çaliskan, 2011; Dolphin et al., 2019). They are also more accessible for students who require increased time flexibility, owing to learning difficulties or mental health requirements (Fletcher et al., 2002; Arrowsmith et al., 2005; Kingsbury et al., 2020), and they cater for those with other time commitments such as part-time work or childcare.
2.2 Disadvantages of VFTs
Several disadvantages are routinely recited including a loss in social cohesion (Butler, 2008; Dunphy and Spellman, 2009), as individuals are typically unable to interact with peers and staff in an informal and flexible manner (Hurst, 1998). Within a virtual context, the experience of travel, outdoors and nature is lost (Bellan and Scheurman, 1998), and sensations such as sound and smell are absent (Hurst, 1998). Embodiment is key within fieldwork (Clark and Jones, 2011; Mogk and Goodwin, 2012), which may be difficult to achieve with a VFT as an individual may not relate the scale of the landscape to their own body (Hurst, 1998). However, embodiment can be improved with 360∘ photo spheres and immersive headsets (Klippel et al., 2019). Certain aspects of traditional field training are difficult to replicate (Hurst, 1998; Arrowsmith et al., 2005) such as the use of a compass clinometer. As VFTs are typically computer based, IT issues can be a concern to many who may not have equal access to computers and the internet (Cliffe, 2017). Furthermore, the increased cognitive load associated with learning new software during a VFT has the potential to detract from the desired learning outcomes (Petersen et al., 2020).
Ultimately, there are numerous reservations about the ability of VFTs to replicate the cognitive, affective and psychomotor skills acquired during traditional fieldwork (Bloom, 1965; Mogk and Goodwin, 2012; Arrowsmith et al., 2005).
3.1 Initial learning objectives
Prior to travel restrictions in response to the COVID-19 pandemic, the two field trips in this study were run to provide field experience covering a wide range of geological aspects required for a broad training in petroleum geoscience. The trips were designed to complement each other and “bookend” the 1-year MSc programme. In a typical year, the Pyrenees trip ran near the start of the academic year (October), and the Utah trip came at the end of the taught component of the MSc course in April. The Pyrenees trip deals with compressional tectonics, foreland basins, carbonate sedimentology and deep-water clastic depositional systems. The Utah trip covers extensional tectonics; rift basins; salt tectonics; fluvial, aeolian, and shallow marine depositional systems; and igneous rocks in a petroleum context. Both courses use a series of exercises that draw on the observations in the field to simulate petroleum exploration and production scenarios. Students typically work in groups (four to six individuals) and present results back to the course tutors and the rest of the class. The importance of teamwork was emphasized to the students, and this was continued in the VFT, as recommended by Arrowsmith et al. (2005) and Dolphin et al. (2019). The goals of the VFTs were to recreate the format of the traditional trips and to achieve the same learning outcomes.
3.2 Student learning outcomes and assessment deliverables – Pyrenees field trip and VFT
The first trip in the academic year was based on data from the Spanish Pyrenees. The VFT was a direct, real-time replacement for the traditional trip, with the same student learning outcomes. On completion of the field trip or VFT students should be able to understand the following:
-
the fundamentals of compressional tectonics and how they relate to the formation of foreland basins;
-
the formation of compressional traps;
-
depositional models for deep water, slope, and basin floor turbidite systems and how they impact heterogeneity in reservoirs;
-
depositional models for carbonate systems within a tectonically active foreland basin;
-
the interaction of tectonics and sedimentation in a compressional setting;
-
the formation and fill of structurally controlled mini basins and the 3D variability of basin fill;
-
petroleum system and play mapping;
-
the structural and stratigraphic evolution of the south Pyrenean foreland basin.
The deliverables were the following:
-
a group presentation detailing the petroleum perspectivity of the study area, including common risk segment maps for a variety of play types;
-
a recommendation for future exploration activity;
-
a compilation (Facies Atlas) of sedimentary geobodies that could form potential fluid reservoirs (for hydrocarbons, carbon capture and storage – CCS, aquifers, etc.), that summarizes their diagnostic criteria, sedimentary structures, dimensions, petrophysical properties and relationship to surrounding deposits (examples of architectural elements include mouth bar, channel bodies, aeolian dunes, etc.)
3.3 Student learning outcomes – Utah field trip and VFT
The second trip of the academic year is to Utah. The virtual version of the trip ran twice, because lockdown occurred mid-way through the academic year; learning outcomes and deliverables include the same student learning outcomes as the traditional trip. On completion of the VFT students should be proficient in understanding the following:
-
depositional systems in rift basins with special reference to the key elements of petroleum systems;
-
sequence stratigraphy of shallow marine and paralic depositional systems, including understanding the importance of depositional process in controlling reservoir architecture and distribution;
-
field development planning in a shoreface/estuarine depositional system;
-
the distributive fluvial system (DFS) model as a predictive exploration tool in fluvial systems;
-
identification and the significance of large sandstone-dominated meander-belt systems;
-
how intrusive igneous can effect petroleum systems, with analogues for the West of Shetland area and the Norwegian Atlantic margin;
-
salt-related fluvial systems and the interplay of depositional systems and changes in accommodation;
-
extensional tectonics and relationships between zones of fault interaction and their reservoir impacts;
-
the geological evolution of central Utah from the Permian to the present day.
The deliverables were the following:
-
an exploration play summary exercise, including group presentations on the plays and perspectivity of the Salt Lake Basin;
-
a field development plan for an estuarine and shoreface reservoir system;
-
prospect evaluation exercise for the salt-related fluvial systems in the Chinle Formation;
-
an evaluation of the key exploration plays in the Salt Valley Anticline area, including integrating seismic, well, and outcrop data, in order to produce a full economic evaluation and recommendation for drilling wells;
-
continued work on the Facies Atlas of sedimentary geobodies started in the Pyrenees.
3.4 VFT planning
After the cancellation of the Utah 2020 April field trip, the members of staff responsible for the course met to discuss alternatives. Given the prior experience in virtual outcrop geology and the access to public (https://v3geo.com/, last access: 4 September 2021; Buckley et al., 2022) and proprietary (https://safaridb.com, last access: 4 September 2021) datasets of over 600 virtual outcrops, the LIME software (Buckley et al., 2019) was chosen to develop and deliver the VFTs. LIME has a direct link to both of these cloud-based databases, along with virtual outcrops to be accessed and shared without the need to copy very large volumes of data (Buckley et al., 2022).
The process of building a VFT is summarized in Fig. 2 and can be broken down into the following stages:
-
VFT scope – decide on desired learning outcomes of the field trip and determine whether the VFT is thematic or location based. The VFTs described within this study are all based directly on previous field trips and are, therefore, location based.
-
build a storyboard – decide on the narrative of the trip using the learning outcomes. Agree on the type and volume of data required. Data include virtual outcrops, sub-regional DEMs (i.e. 10×10 km to provide spatial context for virtual outcrops), figures, traditional field data, subsurface data, photos, satellite images, video clips, 360∘ photo spheres, and links to external resources such as gigapixel panoramic images, videos, and Google Street View. An example template of a storyboard is shown in Fig. 3.
-
compile data – sort internal resources into folders or upload online to reduce file size, such as videos. Compile Uniform Resource Locators (URL) of external resources such as Google Earth Engine in a database such as a spreadsheet (or saved web browser) for future reference. Unify coordinate systems for the spatial/georeferenced data.
-
build the VFT using LIME – separate projects were compiled for each day. A summary of the data used is provided in Table 2.
-
distribute LIME to participants – either as a locally installed software on their personal computers or accessed via the VDI (virtual desktop infrastructure) at the university.
-
distribute the LIME files and supporting material – supporting material includes the field guide, worksheets and maps. In this case, files were uploaded the day before each day of the trip within Blackboard Learn (https://abdn.blackboard.com/, last access: 5 September 2021).
-
assess the effectiveness of the VFT – assess effectiveness throughout the VFT with regular end-of-day discussions, perform after-action review at end of the VFT and run questionnaires for student feedback. Using the acquired assessment, student feedback and staff experience, improve the VFT.
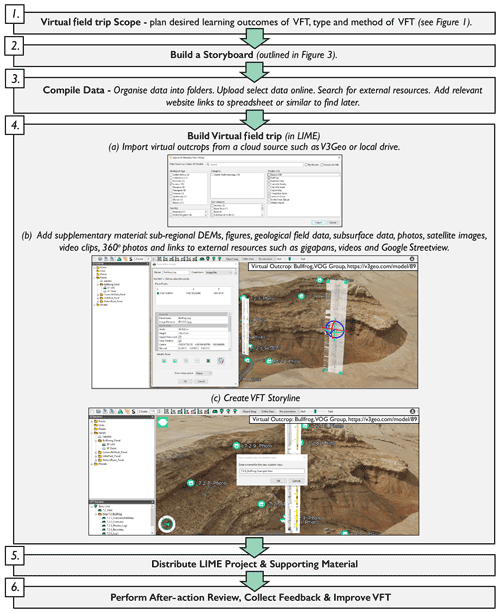
Figure 2Proposed workflow for building and running VFTs, this workflow was followed for every VFT within this contribution.
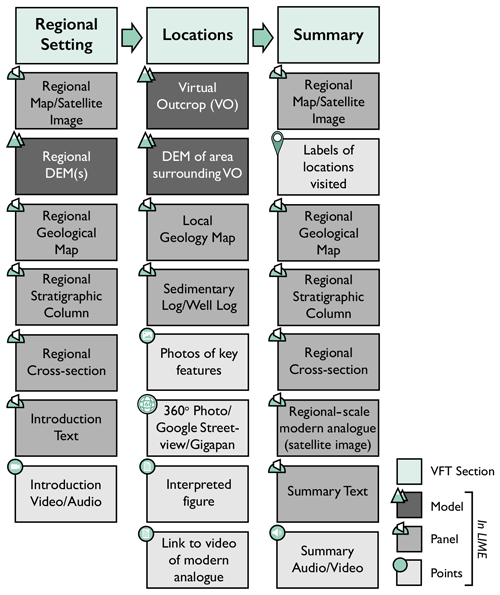
Figure 3Template storyboard for designing a VFT. Data input within LIME is indicated. Locations varied across each day of each trip, from as few as three locations to as many as eight depending on the days' aims. Typically, a new location was created for each new virtual outcrop.
Across both VFTs, there were 2 d where there was insufficient virtual outcrop data at a large enough regional scale to meet the learning outcomes, so Google Earth was used instead of LIME. Building the VFTs took a total of 2 months for the Utah trip and about a month for the Pyrenees VFT; four staff members divided the workload.
3.5 Demographic and setting
All students were enrolled in the GL5013 course “Professional Skills incorporating International Field Trip” of the “Integrated Petroleum Geoscience” (IPG) MSc programme. Table 1 outlines the demographic and setting of students that attended the VFTs. Prior to the COVID-19 pandemic, the 2019–2020 class attended the Pyrenees as a traditional field trip. However, as a direct result of the COVID-19 pandemic many students returned home, and the whole class joined the Utah VFT remotely in September 2020. For the class of 2020–2021, both trips were run as VFTs in October 2020 and August 2021. The relaxations in COVID-19-related restrictions allowed some students to attend these trips from on-campus computer rooms while others worked from home (Table 1). In both cases, the course leaders were remote. Across the three VFTs a general trend of improved average WiFi speeds was observed. WiFi speeds were monitored by staff through Blackboard Learn, and students that had poor internet were offered a free wireless internet dongle for the duration of the VFT; however, no participants accepted the offer. Generally, there were few internet-related issues.
4.1 3D software: LIME
The main software that was used for building and delivering virtual field trips was LIME (Buckley et al., 2019). LIME is a high-performance, lightweight 3D software for visualizing, interpreting, and presenting 3D models and associated data (Buckley et al., 2019). The LIME 2.2.2 version of the software was used for all VFTs, and it was the newest version at the time. LIME was originally created as a simple-to-use software for geoscience applications primarily for navigating, measuring and interpreting large lidar-derived virtual outcrops (Buckley et al., 2019). The rapid expansion of virtual outcrop geology amplified demand for 3D software tailored to geoscience (Buckley et al., 2019), and over the past decade LIME has advanced to facilitate co-visualization of a wide range of data types in addition to virtual outcrops. Such supplementary spatial and non-spatial data include the following:
-
3D models include virtual outcrops and other 3D models such as DEMs, hand samples and models commonly used as scales (e.g. car, human or 10 m measuring pole).
-
Lines are for interpretation lines, mapping contacts and measuring distances.
-
Planes are for correlation and extrapolation of surfaces away from the virtual outcrops and for measuring strike and dip.
-
Panels are 2D planes in 3D space onto which image files can be draped. They serve as “billboards” in the virtual space. They can be used for maps, cross sections, subsurface data, satellite images, explanatory figures, and a host of other uses in the VFT.
-
Points are 1D pins in 3D space. They can be used as place markers, and they can also be used as hot links to launch other material. That material can be internal data (such as photos, figures, videos, or audio that are stored locally within the project) or external data (such as gigapixel panoramic images, 360∘ photo spheres, Google Earth Engine, Google Street View, YouTube, etc.) that are accessed via links from the internet.
LIME allows users to store “custom views” and compile them into a storyline, which allows the course leader to build a narrative through the VFT. The VFT storyline functionality, used extensively in this contribution, enabled preassigned views and animation paths. The views enabled the display of chosen material (models, lines, panels, points and planes) to be stored and accessed in order. The VFT storyline works as a guide for both staff and students when presenting or exploring within LIME. Students navigate between views, ensuring a consistent and streamlined learning experience, through the display of specified material. Each view is created prior to the VFT with chosen models and supplementary material, providing participants with a virtual replacement of locality stops, as well as regional context of those stops.
A typical virtual outcrop will contain around 0.5–1 GB of data, and a typical 1 d virtual field trip may contain five or more virtual outcrops and could easily require >5 GB of disc space. This is prohibitively large for downloading and storing for most students (and users in general). To reduce file and transfer sizes, all virtual outcrops were first converted to multi-resolution tiled models and stored in the cloud at https://v3geo.com/ (last access: 4 September 2021) or https://safaridb.com (last access: 4 September 2021). When building the virtual field trip, they are imported into LIME via the “Import From Cloud Source” function. This ensures that only the data that are required for a specific view are downloaded, and this happens in real time whilst viewing (Buckley et al., 2019, 2022). V3Geo (https://v3geo.com/, last access: 4 September 2021) is a public repository of virtual outcrop data (Buckley et al., 2022), and SafariDB (https://safaridb.com/home, last access: 4 September 2021) is a proprietary database using a similar application programming interface (API). Students were given access to both databases of outcrop analogue data (Howell et al., 2014). The result is that the LIME project folder that is distributed to the students only contains the “other data” (points, lines and images) and is typically a few tens of megabytes in size, which is manageable for the students to download and store.
4.2 Additional software: Google Earth Pro
Since the launch of Google Earth in 2005, it has been regarded as a powerful resource for teaching and research (Lisle, 2006) and has been used within the curriculum of many universities (e.g. Whitmeyer et al., 2009; Monet and Greene, 2012; Giorgis, 2015; Rotzien et al., 2021). Google Earth Pro, the desktop version, allows users to run the application from their own computer, provided they have the minimum system requirement of 2 GB of RAM. Given the integral role Google Earth and Google Earth Pro play within many geoscience degrees, the onset of the COVID-19 pandemic led many universities to partially or fully replace their field trips with Google Earth-based alternatives (e.g. Evelpidou et al., 2021; Bosch, 2021).
The VFTs presented here included days run within Google Earth Pro. On days in which very large areas were studied, such as the first day of Utah, Google Earth Pro provided a regional overview to tasks set. Additionally, certain tools within Google Earth Pro were used, including the “Show Elevation Profile” on a delineated path, offering a cross-sectional profile of the topography. This tool provided an immediate foundation to cross-section construction or discussion.
A limitation of Google Earth is that the imagery is derived through nadir satellite photography (taken from overhead looking vertically down) and is draped onto a DEM of varying resolution (typically 30 m). This results in cliff lines and outcrops being poorly rendered and smeared (Yu and Gong, 2011). This is partially mitigated where Google has integrated additional data in the form of “3D buildings”. This data layer has been gradually implemented since 2012 and uses data from low-angle aerial photogrammetry to provide additional detail of vertical features. It is primarily applied to cities but is increasingly being implemented in areas of “public interest” such as national parks (Google, 2021); therefore, coverage of geological interest areas is typically limited. Despite this, image quality and 3D rendering are very good, and the layer provides an excellent alternative to virtual outcrops if they are not available, although they are not suitable for making measurements or mapping on to.
4.3 Delivery platform: Blackboard Learn
For the past 12 years, Blackboard Learn has been employed as the digital learning platform at the University of Aberdeen. Pre-COVID-19, Blackboard Learn was primarily used for file sharing and assessment submission; however, in March 2020 it became the primary platform to run live lectures and practicals within the “Virtual Classroom”. The virtual classroom also provided a record function, which enabled all days to be recorded for inclusivity of students. Blackboard Learn offers a host of teaching tools including breakout groups for participant interaction, polling to enhance engagement (see Fig. 4), file sharing, and a whiteboard for annotated sketches and discussions. Blackboard Learn also rates the WiFi quality of attendees, providing a visual and numerical indication of individuals who may be experiencing poor connection. All students and staff had access and familiarity with Blackboard Learn; therefore, it was selected as a platform to run the VFTs.
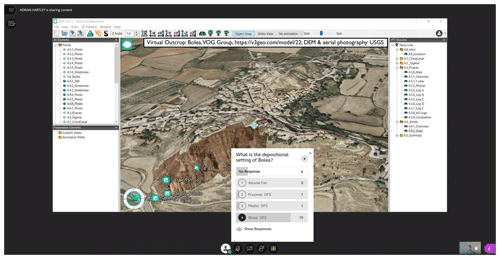
Figure 4Bolea, Aragon, Spain; example of a poll being run within Blackboard Learn virtual classroom; students were asked to identify the depositional setting of the locality. Virtual outcrop: Bolea, VOG Group, https://v3geo.com/model/22 (last access: 4 September 2021); aerial photography on DEM, USGS EROS Archive; DEM, USGS 3D Elevation Program.
4.4 Virtual desktop infrastructure
LIME (and to a lesser extent Google Earth) requires PCs with a moderate to good processing power and moderate graphics capabilities. Whilst these are typically available for industrial/commercial consumers of VFTs, this is often not the case for all students. The University of Aberdeen runs a Virtual Desk Infrastructure (VDI) which allows students and staff to remotely log in to a virtual computer in the university. That way, the processing is handled on the virtual machine and the student's computer acts as a terminal. This allows students with low-grade computers or computers that do not support the software (e.g. Mac) to run all the required software. With a reasonable internet speed (>6 Mbps), there is only a short lag time and the system worked well.
Prior to running each trip, a document detailing how to operate the software used was digitally distributed to the participants (Supplement S1) along with a digital version of the field guide. Material for each day of the trip was typically made available the evening before. Students were advised to download the files prior to the start of the VFT to prevent Blackboard Learn connection issues and to ensure that they would not be negatively affected by slow download speeds. At the start of each day, a poll was conducted to gauge whether all students had been able to download and open the material for that day. Any software issues were resolved by a staff member ahead of assessments. All assessments, except for the facies atlas, were based on group work. The students were allocated groups via a group list uploaded to Blackboard Learn. Groups were allocated by the same process as traditional field trips, i.e. at random with some minor modifications to ensure a spread of ability and diversity.
5.1 Utah VFT: outline
Direct replacement of a traditional field trip lasted 11 (2020) and 10 (2021) days. A separate LIME project was built for most of the days. The Utah 2020 data are outlined in Table 2a, with minor improvements made to most days on the Utah 2021 VFT based on the after-action review and student feedback. The days that changed significantly between 2020 and 2021 are outlined in Table 2b. Over the Utah VFTs, an average of 30 virtual outcrops, 16 DEMs, 318 photos, 101 logs/wells and many other data were used (see Table 2 and Fig. 5). The volume of material provided to the students was well received, and staff were confident that most students used the material to emulate the practices undertaken on traditional fieldwork (example shown in Fig. 5). Supplement S2 provides a list of publicly accessible V3Geo virtual outcrops used during both VFTs.
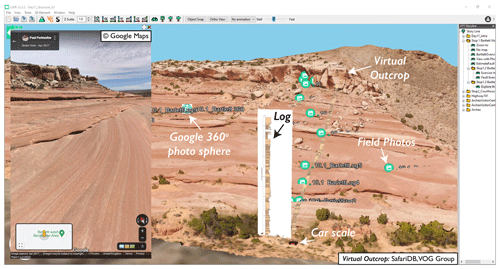
Figure 5Bartlett Wash, Utah, USA. The task at this locality is to interpret the depositional environment and consider reservoir compartmentalization. Virtual outcrop imported from SafariDB (2021; collected and processed by VOG Group) and viewed in LIME with additional material including logs, photos and 360∘ photo spheres (example inset © Google Maps).
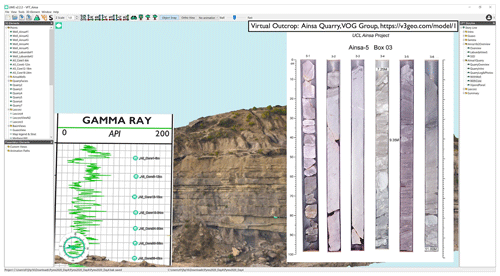
Figure 6Ainsa Quarry, Spain, a view within the Pyrenees 2020 virtual field trip. Virtual outcrop data are available at the following: Ainsa Quarry, VOG Group, https://v3geo.com/model/1 (last access: 1 September 2021), supplemented with additional material including well logs and core photos within LIME. Core photos from Pickering and Corregidor (2005).
Days 1 and 2 of the Utah VFT examined modern basin and range tectonics around the Great Salt Lake, which were run from Google Earth Pro and culminated in an exploration play mapping exercise. Day 3 centred around the northern Book Cliffs and focused on shallow marine sedimentology (shorefaces and river-dominated deltas) and sequence stratigraphy (Howell and Flint, 2003; Enge et al., 2010; Enge and Howell, 2010). Day 4 was focused further south in the Book Cliffs, in Woodside Canyon, and examined a tidal estuarine package interpreted as an incised valley complex (Howell and Flint, 2003; Sømme et al., 2008; Howell et al., 2018). The VFT continued south in the Book Cliffs for day 5, focussing on sequence stratigraphy and correlation in shoreface parasequences within the section directly north of the town of Green River in the morning (Pattison, 1995; Jackson et al., 2009; Eide et al., 2015). The afternoon of day 5 was spent at Thompson Canyon (Van Wagoner, 1995), where the students completed a field development exercise. All Book Cliffs days used a series of large-scale (kilometres) virtual outcrops and were run within LIME.
For day 6, the field trip looked at older Cretaceous stratigraphy along the western side of the San Rafael Swell, including the transgressive deposits of the Dakota/Naturita system (Phillips et al., 2020) and the growth faulted, fluvial-dominated deltas of the Ferron Sandstone (Bhattacharya and Davies, 2001; Enge et al., 2010; Braathen et al., 2018). Day 7 compared the fluvial architecture of the incised Shinarump Sandstone at Capitol Reef with the distributive fluvial deposits of the Salt Wash Member of the Morrison (Owen et al., 2015). Special reference was made to the recognition of sand-dominated meandering systems, such as the large meander belt exposed at Caineville Wash and the Notom Road localities (Hartley et al., 2015).
Day 8 discussed igneous–sedimentary interactions of the Caineville and Henry Mountain area (Horsman et al., 2005). Day 9 included a traverse through Canyonlands, reviewing the stratigraphy and comparing different types of arid continental reservoirs. Day 10 was a detailed study of the interaction between the salt tectonics related to the Paradox formation evaporites and sedimentation within the Chinle Formation fluvial deposits (Matthews et al., 2004; Hartley and Evenstar, 2018). Day 10 culminated in a major student exercise dealing with exploration in salt basins. The final day, day 11, focused on extensional tectonics around Moab and within Arches National Park. The students visited a series of localities along the Moab Fault (Foxford et al., 1998) and at the Delicate Arch Relay Ramp (Rotevatn et al., 2009).
Utah 2021 followed a similar outline with minor changes to most days and slight changes to the running order. Three days changed considerably. For days 1 and 2, the “Rift basins and exploration” components around Salt Lake were combined into a single day, covering the same volume of material. The “Salt-related systems” day was moved into LIME rather than using Google Earth Pro, because LIME enabled better presentation of the additional data such as the sedimentary logs. The final day was a new assessed exploration exercise, which combined outcrops with seismic and well data from the Salt Valley anticline. This replaced the Canyonlands day. This exercise used LIME to combine the subsurface data with the outcrops.
5.2 Pyrenees VFT: outline
Days 1 to 5 of the Pyrenees VFT included 30 virtual outcrops, 20 DEMs, 92 photos, 20 logs/wells and other data (see Table 2c). Again, the amount of material provided to the students was well received, and the extensive dataset allowed students to explore the area and apply their geological understanding to a similar extent as a traditional field trip. Day 1 provided a regional geological overview and introduction to the structure of the Pyrenees with a transect from the axial zone to the Jaca Basin along the Hecho Valley. Google Earth Pro was implemented due to the large geographical scale of the day. Day 2 continued the transect south, crossing from the Jaca Basin, through the External Sierras to the Ebro Basin, along the Gallego Gorge. Here, thrust tectonics were combined with an examination of the syntectonic alluvial fans in Riglos and Aguero (Nichols, 1987). In the afternoon of day 2, the trip moved to Arguis and Pico de Aguillar, where lateral changes along the thrust front and the role of salt with the detachment were discussed.
Day 3 considered the distributive fluvial system (DFS) deposits of the Huesca DFS (Nichols and Hirst, 1998) of the Ebro Basin. Day 4 studied the deep-water deposits of the Ainsa Basin, combining the data of wells and cores from directly behind the outcrop (Pickering and Corregidor, 2005) (Fig. 6), with outcrop reservoir models (Falivene et al., 2006) and synthetic seismic data (Bakke et al., 2008). The final day of this VFT, day 5, was an assessed group exercise, reviewing the prospectivity of the south Pyrenean Foreland Basin, and it required the students to revisit all the stops we had visited previously. A summary of the VFT was presented to the students within LIME after the student group presentations on basin evaluation.
6.1 Student experience
Student experience was evaluated through two different questionnaires. Ethics approval was granted for all questionnaires by the University of Aberdeen. The university provides a standard form (Course Evaluation Form) that is completed after every course. These were used as they provide a benchmark to compare the VFT with the physical course over the previous 5 years. In addition, a specific questionnaire was conducted to provide a more detailed, day-by-day insight into the VFTs. In these, questionnaire participation was voluntary and anonymized, under university guidelines. Individuals answered a series of questions rating their experience between 1 (disagree) to 5 (agree) and were provided with the opportunity to give qualitative statements to provide further information to their answers within an open text box. Supplement S3 is an example of one of these; the same format was used for all three VFTs. On the Utah 2020 VFT there was a questionnaire response of 88 % (24 out of 27); for Pyrenees 2020 it was 100 % (23 out of 23), and for Utah 2021 it was 90 % (19 out of 21). Students were asked to fill out the questionnaire on the final day of each VFT, with extra time allocated to a break; individuals who wished to respond after the VFT were asked to do so within 2 weeks. Individuals that were unable to attend the full field trip due to other commitments did not answer the questionnaire; this included five in Utah 2020 and two in Pyrenees 2020. A total of 66 questionnaire responses were collected.
Standardized course evaluations and questionnaires are routinely used across the academic curriculum to gauge student perception. Nonetheless, the authors acknowledge there is an issue of self-reporting (Spooren et al., 2013; Boring and Ottoboni, 2016; Esarey and Valdes, 2020). Students can only draw on their own experience and are unable to truly compare between a traditional field trip and a VFT if they do not attend both. Furthermore, the notion of understanding is not a true measure of understanding, as an individual cannot evaluate the true extent of what they understand (Kuorikoski and Ylikoski, 2015). However, all students had attended a traditional field trip at some point in their education; therefore, each had field experience to base their opinions on. As there is not a way to truly standardize the data, the questionnaires presented are used to gauge general opinions and suggested improvements.
6.2 Activity and attendance
Within Blackboard Learn, auto-generated reports are accessible through the Evaluation and Course Reports functions. These reports provide insights into usage and student activity across Blackboard Learn. The data include the overall time an individual spent within the course, as well as information about their activity within the content area, from time spent to number of accesses. Each day of the VFT was also allocated its own virtual classroom, allowing reports to be run for every day assessing student attendance across the VFT. These reports were accessible to staff as a Microsoft Excel file or comma separated value (CSV) file, and the relevant data were extracted.
6.3 Duration analysis
Over the Utah 2021 VFT, activities in each day were divided into categories and timed using a digital stopwatch. The total active time within 9 d was 43 h, 40 min and 8 s, with an additional 14 h, 22 min and 11 s of allocated breaks (e.g. lunch). Day 10 was not timed due to a change in plans related to a COVID-19 incident in the shared computer room.
6.4 After-action review
On completion of a VFT, the staff and demonstrators discussed what they felt had worked and what could be improved across the VFTs. After-action reviews took place at the end of most days and at the end of each field trip. Suggestions from this after-action review and free-text comments were noted for the Utah 2020 VFT, and where appropriate, enhancements were implemented prior to Utah 2021.
Questionnaires were compiled, free-text comments were added to a master Microsoft Word document and numerical answers were summed in a master Excel spreadsheet. Later trips were added to the same master documents, facilitating direct comparisons to be made. Numerical responses across the three trips were plotted and compared through box-and-whisker plots, giving the range in responses for each trip. Blackboard Learn evaluations and course reports were compiled, selecting relevant information. Duration analysis was evaluated and averaged.
7.1 Course evaluation forms
The course evaluation forms provided a valuable comparison between student feedback for before and during the COVID-19 pandemic. The two course evaluation forms from 2016–2017 and 2017–2018 are when both trips were traditional field trips. The 2018–2019 results were unfortunately not available for analysis due to controls outside this study. The 2019–2020 form evaluates the year when the Pyrenees trip ran as a traditional field trip, whereas Utah ran as a VFT due to the onset of the COVID-19 pandemic. The form from 2020–2021 represents the year in which both trips ran as VFTs due to the COVID-19 pandemic.
Across the three questions within the course evaluation forms, a notable improvement in results is observed between the oldest (2016–2017) and most recent (2020–2021). With 100 % of 2020–2021 students totally agreeing that they enjoyed (Fig. 7b) the course, and it improved their graduate attributes/employability (Fig. 7c). The 2020–2021 students also all agreed that the teaching was effective, with 87.5 % (Fig. 7a, the highest of all 4 years) totally agreeing and the remaining 12.5 % agreeing. Again, while standardized course evaluations can be regarded as unreliable (Boring and Ottoboni, 2016; Esarey and Valdes, 2020; Spooren et al., 2013), this does not undermine the overwhelmingly positive perceptions of the students attending the VFTs. Although no precise comparisons can be made between the traditional field trips and VFTs due to a change in student cohorts, the data illustrate that students appear generally satisfied with the VFTs.
7.2 General learning outcomes
The questionnaire results for general learning outcomes are presented in Fig. 8a–b. Across all three field trips an average of 94.3 % of students agreed that they “had learnt new things during the VFTs”, with the remaining 5.7 % scoring neutral; no student disagreed, and interquartile ranges (IQRs) were all plotted between 4 and 5. The overarching learning outcome statement of “I have a better understanding of exploration processes” was rated mostly positive for the Utah VFTs with IQRs between 4 and 5; for Utah 2020 91.7 % of students agreed, and for Utah 2021 it was 89.5 %. The Pyrenees IQR had a wider range from 3–5, with an average of 73.9 % of students agreeing.
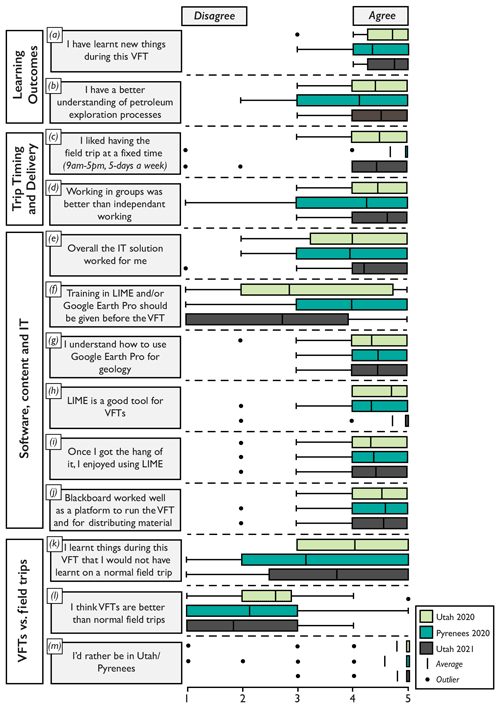
Figure 8Quantitative responses to questionnaires for all three VFTs presented as box-and-whisker plots. Panels (a) and (b) summarize learning outcomes; panels (e) to (j) summarize software, content and IT; and panels (k) to (m) summarize comparative statements between VFTs and traditional field trips. Responses are collated for each trip presented for comparison.
7.3 Trip timing and delivery
The statement “I liked having the field trip at a fixed time” (Fig. 8c) scored positively across all three trips, with the Pyrenees VFT participants responding particularly positively (95.7 %), with an IQR of 5. For the Pyrenees 2020 VFT, there was one individual who responded negatively, and the Utah 2021 VFT had two individuals who disagreed. Students across all three VFTs mostly agreed that “working in groups was better than independent working” (Fig. 8d). Both Utah VFTs received particularly positive responses, with the 2020 VFT scoring 83.3 % and the 2021 VFT scoring 94.7 %, and remaining scores on both VFTs were neutral. The Pyrenees 2020 VFT, in contrast, exhibits a broader IQR between 3 and 5, with 4.3 % disagreeing, 26.1 % scoring neutral and 69.6 % positive.
The daily average time that students spent within the virtual classroom across the Utah 2020 VFT was 5 h and 49 min; for the Pyrenees it was 6 h and 22 min, and for Utah 2021 it was 6 h and 48 min. A breakdown of average time spent doing activities is illustrated in Fig. 9. Group work tasks (23 %), LIME-guided VFT (21 %), independent work in LIME exploring the virtual outcrop and supplementary data (8 %), and discussions (7 %) formed a large portion of the work activities during the VFT and emulated similar activities of traditional fieldwork. A very small portion of the VFT was spent providing technical instruction of software, outlining assessment and presentation of external material, such as Google Earth Engine. Time spent waiting, which includes waiting for students to rejoin after lunch, share screen and resolve technical issue also formed a very small proportion of the trip at an average of 3 % of each day.
7.4 Software, content and IT
IT solutions worked for most participants across all VFTs (Fig. 8e). The Utah 2020 VFT had a 75 % positive response (IQR between 3.25 and 5); for Pyrenees 2020, 69.6 % of responses were positive (IQR between 3 and 5), and for Utah 2021 a higher 84.2 % of students responded positively (IQR between 4 and 5). There were occasions when IT solutions did not work for individuals, such as for Utah 2021 where an individual scored 1. However, this individual's WiFi was negatively impacted by unexpected local issues that were beyond the control of staff.
The statement “Training in LIME and/or Google Earth Pro should be given before the VFT” (would require an extra day) (Fig. 8f) was met with a full range of responses and wide variations in IQRs. The two VFTs (Utah 2020 and Pyrenees 2020) where most students had the highest agreeing response, with 37.5 % of Utah 2020 and 65.2 % of Pyrenees 2020 participants, indicating they would have preferred a day of software training prior to the VFT. Although, for the Utah 2021 VFT individuals had already attended the Pyrenees 2020 VFT, and 52.6 % of individuals disagreed with the statement. Scores were consistent for the statement “I understand how to use Google Earth Pro for geology” (Fig. 8g), with over 80 % agreeing across all VFTs.
LIME scored positively as “a good tool for VFTs” across all three VFTs (Fig. 8h); 100 % of the Utah 2020 VFT agreed, as did 78.3 % of the Pyrenees 2020 VFT and 94.7 % of the Utah 2021 VFT. Two individuals across the three VFTs disagreed in both cases they were individuals who reported lower WiFi speeds, which were unable to be resolved in the VFT time frame. The same year group who joined the Pyrenees 2020 and Utah 2021 VFT displayed a positive shift in perceptions between the two VFTs in the view of LIME as a VFT tool. The statement regarding individuals who enjoyed LIME after they became more familiar with the platform (polled as “Once they got the hang of it”) was also met with a mostly positive and markedly consistent response across all three VFTs. Interquartile ranges (IQRs) were consistent, falling between 4 and 5, with an average of 86.5 % agreeing, 5.8 % neutral and 3.2 % disagreeing. As a “platform to run the VFT”, most agreed that “Blackboard worked well” and with a consistent response across all VFTs which presented IQRs spanning 4–5 (Fig. 8j).
7.5 Virtual field trips and traditional field trips
Responses for the statement “I learnt things during the VFT that I would not have learnt on a normal field trip” were diverse. The Utah 2020 VFT perceptions were predominately positive with 66.7 % of students scoring between 4 and 5 and 33.3 % were neutral, with no students disagreeing. The Pyrenees 2020 VFT presented a larger IQR range of 2–5; there 34.8 % of students agreed, 30.4 % were neutral and 34.8 % of students disagreed. The Utah 2021 VFT also offered a large IQR range of 2.5 to 5; there 57.9 % of students agreed, 15.8 % were neutral and 26.3 % of students disagreed.
With the statement “I think VFTs are better than normal field trips” (Fig. 8l), there was a broad, predominately negative response with IQRs spanning 2–3 and 1–3, most did not agree that VFTs were better. When asked if they would rather be in Utah/Pyrenees, all IQRs fell at 5 (Fig. 8m), with medians falling between 4.6 and 4.8. An average of 92.4 % of students would rather be in the field, although there were some outliers. Within the free-text comments, individuals who preferred the VFT stated cost of fieldwork and accessibility as the main reasons for their scores.
7.6 Individual days
Each VFT day was listed across all three VFTs and students were asked to score the statements “I learnt a lot from this day” and “I enjoyed this day”. For assessed days, they were asked if the “exercise worked well”.
7.6.1 Results for Utah 2020 VFT
Both for learning and enjoyment this VFT was scored positively and consistently by participants (Fig. 10 a and b). Ten of the day's IQRs fell between 4 and 5 for learning, with day 11 as the exception with an IQR between 4 and 4.75. Enjoyment IQRs were a consistent 4–5. The average positive response across all days of this VFT was a 94.6 % for learning and 90.3 % for enjoyment.
7.6.2 Results for Pyrenees 2020 VFT
This VFT presented a higher range and day-to-day variation. Days 1–2 IQRs sit between 3 and 5 for learning, with the full range of scores represented (Fig. 10c). For enjoyment, day 1 scored higher than learning (Fig. 10d) with an IQR of 3.75–5, whereas day 2 was consistent with the learning IQR. Students were more positive about day 3–4 with IQRs for both increasing to 4–5 for learning. Enjoyment peaked on day 3 with an IQR of 4.5–5. Overall, the average positive responses for the Pyrenees VFT for learning and enjoyment were 79.35 % and 80.29 %, respectively.
7.6.3 Results for Utah 2021 VFT
This VFT scored consistently for both enjoyment and learning presenting IQRs of 4–5 for all days (Fig. 10e and f). The average positive responses for the Utah 2021 VFT for learning and enjoyment were 90.2 % and 87.9 %, respectively, which is a notable increase from the previous Pyrenees VFT that the class attended. This increase is largely attributed to over half the class (57.9 %, Table 1) located on campus, where they were able to interact in person (within social distancing guidelines).
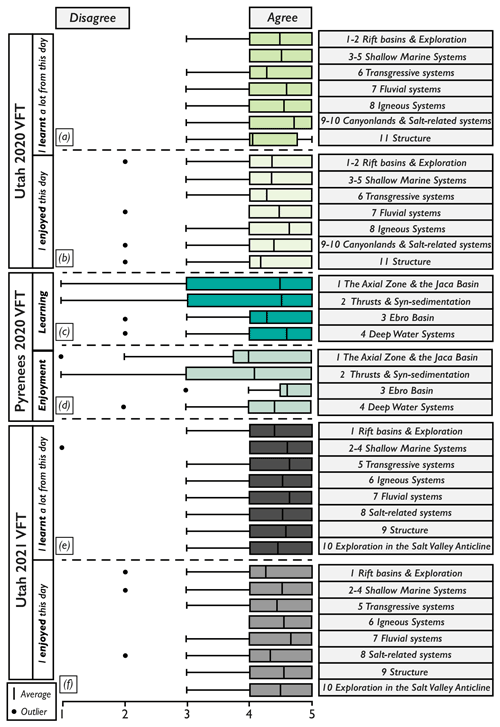
Figure 10Quantitative responses to day learning and enjoyment for all three VFTs presented as box-and-whisker plots. (a, b) The responses to the 11 d of field trip; panels (c) and (d) summarizes software, content, and IT, and panels (k) to (m) summarize comparative statements between VFTs and traditional field trips.
8.1 Interpretation of evaluation
8.1.1 Student course evaluation
Within the student course evaluation, improvements in the student perceptions are observed from pre-COVID-19 to during pandemic learning. This is attributed to a couple of variables. Firstly, the VFTs ran during or around the COVID-19 lockdowns, at a time when all courses operated online. Student moral was lower than a traditional year, and the students verbally expressed low expectations ahead of the VFTs. The free-text comments reflect how the students felt that the VFT was better than they had anticipated, with individuals stating, “overall I thought it was much better than expected”, “above my expectations” and “it was far more engaging than I thought it would be, and I am surprised by how interactive it was”. Secondly, students also acknowledge the quality and extent of the VFTs, with free-text comments including “on the whole the class felt very positive about the trip”, “we were all really impressed by the example that has been set for VFTs”, and “I think as a replacement the VFT was fantastic, very engaging and an exemplary substitute for the field trip”. Finally, students were clearly satisfied with the content of the VFTs and felt they provided an effective teaching experience, which they enjoyed with comments including “I actually thought I learnt more on the virtual field trip as it was easier to understand the context and get my bearings” and “very enjoyable and well organized trip”.
However, it is noted that while the students were positive about their VFT experience, the majority still stated they would have preferred a traditional trip physically travelling to Utah and the Spanish Pyrenees. Many free-text comments reflected this including “although I would have preferred to have been in the Pyrenees, the virtual field trip was still very beneficial”; “I think everyone would much rather be in Utah for the scenery and culture, but I found it easier to focus and understand on the VFT than normal”, and “I think the field trip had many benefits and I did feel like I learnt a lot, but I would [have] probably preferred being in Utah”. Again, this highlights the issue with standardizing the course evaluation forms, as the improvements in the VFT years compared to pre-COVID-19 traditional field trips do not appear to relate directly to the VFT but instead to the context of COVID-19 and low expectations for VFTs.
8.1.2 Questionnaire
Utah questionnaire results for general learning outcomes illustrate that student attitude was largely positive, and students broadly agreed that they had learnt during the VFTs and developed a better understanding of exploration processes. Staff were also largely satisfied that the more specific learning outcomes had been achieved.
The structure and duration of the course was specifically designed to emulate that of a traditional field trip, which worked for most participants. Students agreed that having a fixed time for the VFT worked, and for those who were absent for short sections, recordings were made available. The students also agreed that working in groups was particularly helpful during online learning and eased the negative impact of those with IT issues due to the screen-share function. The importance of group work noted within this study is in line with others (e.g. Arrowsmith et al., 2005; Stumpf et al., 2008; Atchison and Feig, 2011; Lukes, 2014).
Time spent during the VFTs was used efficiently; there was little wasted time on travel. In the previous, real-world Pyrenees field trip, a diary of time spent illustrated that an average of 3.50 h (max 5.1 and min 2.2) was spent travelling by coach or walking to the outcrop. There is clearly a significant time saving in the VFT, and that time was used on study and exercises.
IT solutions worked for most students, with only a few, mainly WiFi-related, issues encountered. An average of 44.8 % of students felt there was a need for some training within the VFT software prior to the running of the VFTs, indicating this may be a useful addition going forward. Students were largely positive about LIME as a tool for VFTs, with the Utah 2021 VFT being particularly positive, attributed to their developed skills in using LIME over two VFTs. The use of Google Earth and Blackboard Learn were also met with a positive response by the students, but neither showed the improved metrics of LIME.
The Utah days of the field trips were rated consistently across the 2 years of delivery. Pyrenees days showed a higher level of variation; however, a general increase in metrics is observed over the duration of the VFT. The Pyrenees VFT ran during the first term of the masters programme. Due to COVID-19, the students had little peer-to-peer social interaction prior to the VFT, and most had never used LIME before, both possible influences on the lower scoring of days 1–2 of Pyrenees.
Within the students' qualitative free-text comments, a consistent theme of improved 3D visualization and geospatial understanding was often stated for the VFT relative to a traditional field trip. Student comments included “I thought the VFT was much better for the regional context as well as 3D thinking”, “being able to see things in 3D and from a bird's eye view was very useful” and “the ability to manoeuvre through different scales (quickly) and around the outcrop to different angles was excellent and not possible on a normal field trip”. Students also self-reported that they believed the learning outcomes were met, with comments including “definitely think the learning outcomes were achieved” and “I don't think there were many learning objectives that could be hit any better”. However, some students expressed that they believed they would have learnt more on traditional fieldwork, with comments including “VFTs cannot fully replace learning from the field such as small sedimentary structures”. Generally, the free-text comments reflected the students opinion that the VFT is not a better experience than a traditional field trip, which is in line with the quantitative results. However, many stated an integration of the two (VFT and tradition field trip) would be beneficial to their learning.
8.2 Implications for future geological field trips
The COVID-19 global pandemic has increased the demand and interest in VFTs, leading to rapid developments in the creation of virtual field trips to numerous locations globally by a myriad of authors. While traditional field trips remain the foundation of many geology degrees, we argue there is a key role for VFTs beyond COVID-19 for several reasons. Firstly, students self-reported an increased 3D and geospatial understanding within the VFT, compared to their experience of traditional fieldwork. Secondly, it is noted that many of the negative aspects of VFTs have the potential to be significantly mitigated by running VFTs in person within a classroom environment. This is illustrated by the positive increase in learning and enjoyment expressed by the students of the Utah 2021 VFT, where over half the class was able to join on campus from the course's designated computer room. With the whole class and staff located on campus, we would anticipate further improvement in perceptions, facilitated by peer-to-peer and staff–student interactions taking place in person. Additional benefits would include an equal distribution of IT equipment and WiFi speeds, and easier detection of students who require further assistance.
Ultimately, a blend of traditional fieldwork with VFTs, specifically virtual outcrop, would further reduce the disadvantages of VFTs. Other studies have reported similar findings with VFTs implemented as a supplement to traditional fieldwork (Litherland and Stott, 2012; Peat and Taylor, 2005), including a preview and/or preparation to fieldwork, during fieldwork or a post fieldwork overview (Hesthammer et al., 2002; Çaliskan, 2011). Within this contribution, an average of 53.1 % of students agreed that they learnt material during the VFT that they would likely not have learnt during a traditional field trip. This further illustrates the potential scope for future implementation of VFTs, particularly during the likely digital alteration in the global working structure, with the many corporations and businesses encouraging at-home working into the future.
The VFTs presented here provided students the opportunity to observe, interpret and apply their geological understanding to a series of localities using virtual outcrops. The VFTs delivered were a direct replacement of traditional field trips that ran prior to COVID-19. This contribution illustrates experience gained and the value of VFTs as a total replacement for traditional field trips and excursions during a time when travel and social integration was restricted due to the COVID-19 pandemic. A cohesive dataset consisting of multiple virtual outcrops, DEMs, field photos, 360∘ photo spheres, maps, cross sections and schematic diagrams enabled students to implement many of the same skills utilized during traditional field trips. Through the interrogation of student quantitative questionnaire responses, as well as their qualitative free-text comments, we demonstrate that the benefits of VFTs are far reaching, with many highlighted advantages mirroring other researchers' findings. Course evaluation improvements were observed during the VFT years, compared to pre-COVID-19 traditional field trips, albeit in the prism of COVID-19 and lower expectations of the VFT. Students nevertheless enjoyed the VFT, and staff were satisfied that the learning outcomes were achieved.
This study ultimately demonstrates that it is possible to replace a traditional field trip with a VFT addressing the same learning outcomes. However, true emersion within the landscape, culture and physical outdoor environment cannot be fully recreated. We therefore argue that VFTs, with a strong virtual outcrop component, can be integrated with traditional fieldwork to deliver a best-of-both-worlds approach for geological curricula, beyond COVID-19.
Many virtual outcrop models presented in this paper are available on V3Geo (https://v3geo.com/, last access: 4 September 2021; V3Geo, 2021), linked within Supplement S2.
The supplement related to this article is available online at: https://doi.org/10.5194/gc-5-227-2022-supplement.
JAH, AH, NS and JHP developed the virtual field trips. JAH and JHP designed and distributed the questionnaires. JHP and JAH wrote the main article draft. GM and RB aided in the running of the VFTs. MC was responsible for processing most of the model data used across the VFTs. JAH, SJB and NN conceptualized the VFT tools available in LIME, which were implemented by KR, JV, and the LIME team. All authors read and gave input through multiple iterations of the article draft.
The contact author has declared that neither they nor their co-authors have any competing interests.
Publisher’s note: Copernicus Publications remains neutral with regard to jurisdictional claims in published maps and institutional affiliations.
This article is part of the special issue “Virtual geoscience education resources”. It is not associated with a conference.
The authors would like to thank all IPG MSc participants for contributing their perceptions and thoughts on the VFTs. The VOG group is acknowledged for their extensive work on virtual outcrops, as is the LIME team for developing the software and providing support through all VFTs. V3Geo is also acknowledged as a useful cloud-based platform to host many of the 3D models used within the VFTs, and the SAFARI consortium (https://safaridb.com/home, last access: 4 September 2021) is thanked for hosting the remaining virtual outcrops and the support. Harry Johnson, a demonstrator on the first Utah 2020 VFT, is thanked for his participation in the course and assistance in questionnaire design. Finally, we thank the editors John Hillier and Steven Whitmeyer and reviewers Glenn Dolphin, David M. Hodgson, Paul Nesbit and Ryan Petterson for their insight.
This paper was edited by Steven Whitmeyer and reviewed by Paul Nesbit, David M. Hodgson, Glenn Dolphin, and Ryan Petterson.
Argles, T., Minocha, S., and Burden, D.: Virtual field teaching has evolved: Benefits of a 3D gaming environment, Geology Today, 31, 222–226, https://doi.org/10.1111/gto.12116, 2015.
Atchison, C. L. and Feig, A. D.: Theoretical perspectives on constructing experience through alternative field-based learning environments for students with mobility impairments, Qualitative Inquiry in Geoscience Education Research, 44, 11–21, https://doi.org/10.1130/SPE474, 2011.
Bailey, J., Whitmeyer, S., and De Paor, D. (Eds.): Introduction: The application of google geo tools to geoscience education and research, in: Google Earth and Virtual Visualizations in Geoscience Education and Research, Geological Society of America, 492, 7–19, https://doi.org/10.1130/2012.2492(00), 2012.
Bakke, K., Gjelberg, J., and Petersen, S. A.: Compound seismic modelling of the Ainsa II turbidite system, Spain: Application to deep-water channel systems offshore Angola, Mar. Petrol. Geol., 25, 1058–1073, https://doi.org/10.1016/j.marpetgeo.2007.10.009, 2008.
Barth, N. C., Stock, G. M., and Atit, K.: From a virtual field trip to geologically reasoned decisions in Yosemite Valley, Geosci. Commun., 5, 17–28, https://doi.org/5194/gc-5-17-2022, 2022.
Bellan, J. M. and Scheurman, G.: Actual and virtual reality: Making the most of field trips, Soc. Educ., 62, 35–40 1998.
Bellian, J. A., Kerans, C., and Jennette, D. C.: Digital outcrop models: applications of terrestrial scanning lidar technology in stratigraphic modeling, J. Sediment. Res., 75, 166–176, https://doi.org/10.2110/jsr.2005.013, 2005.
Bhattacharya, J. P. and Davies, R. K.: Growth faults at the prodelta to delta-front transition, Cretaceous Ferron sandstone, Utah, Mar. Petrol. Geol., 18, 525–534, https://doi.org/10.1016/S0264-8172(01)00015-0, 2001.
Blackboard Learn: https://abdn.blackboard.com, last access: 5 September 2021.
Bloom, B. S.: Taxonomy of Educational Objectives: The Classification of Educational Goals, 1, 6–12, 1965.
Bond, C. E. and Cawood, A. J.: A role for virtual outcrop models in blended learning - improved 3D thinking, positive perceptions of learning and the potential for greater equality, diversity and inclusivity in geoscience, Geoscience Communication, 4, 233–244, https://doi.org/10.5194/gc-4-233-2021, 2021.
Boring, A. and Ottoboni, K.: Student evaluations of teaching (mostly) do not measure teaching effectiveness, ScienceOpen Research, 11 pp., https://doi.org/10.14293/S2199-1006.1.SOR-EDU.AETBZC.v1, 2016.
Bosch, R.: Development and implementation of virtual field teaching resources: two karst geomorphology modules and three virtual capstone pathways, Geosci. Commun., 4, 329–349, https://doi.org/5194/gc-4-329-2021, 2021.
Braathen, A., Midtkandal, I., Mulrooney, M. J., Appleyard, T. R., Haile, B. G., and Van Yperen, A. E.: Growth-faults from delta collapse–structural and sedimentological investigation of the Last Chance delta, Ferron Sandstone, Utah, Basin Res., 30, 688–707, https://doi.org/10.1111/bre.12271, 2018.
Buckley, S. J., Howell, J. A., Enge, H. D., and Kurz, T. H.: Terrestrial laser scanning in geology: Data acquisition, processing and accuracy considerations, Journal of the Geological Society, 165, 625–638, https://doi.org/10.1144/0016-76492007-100, 2008.
Buckley, S. J., Kurz, T. H., Howell, J. A., and Schneider, D.: Terrestrial lidar and hyperspectral data fusion products for geological outcrop analysis, Comput. Geosci., 54, 249–258, https://doi.org/10.1016/j.cageo.2013.01.018, 2013.
Buckley, S. J., Kurz, T. H., Jaboyedoff, M., Derron, M.-H., and Chandler, J. H.: Virtual Geoscience Conference 2016: Where geomatics meets geoscience, Photogramm. Rec., 32, 346–349, https://doi.org/10.1111/phor.12220, 2017.
Buckley, S. J., Ringdal, K., Naumann, N., Dolva, B., Kurz, T. H., Howell, J. A.: and Dewez, T. J.: LIME: Software for 3-D visualization, interpretation, and communication of virtual geoscience models, Geosphere, 15, 222–235, https://doi.org/10.1130/GES02002.1, 2019.
Buckley, S. J., Howell, J. A., Naumann, N., Lewis, C., Chmielewska, M., Ringdal, K., Vanbiervliet, J., Tong, B., Mulelid-Tynes, O. S., Foster, D., Maxwell, G., and Pugsley, J.: V3Geo: a cloud-based repository for virtual 3D models in geoscience, Geosci. Commun., 5, 67–82, https://doi.org/5194/gc-5-67-2022, 2022.
Butler, R.: Teaching geoscience through fieldwork. Higher Education Academy Subject Centre for Geography, Earth and Environmental Sciences, 17–22. ISBN 1-84102-1458-8, 2008.
Çaliskan, O.: Virtual field trips in education of earth and environmental sciences, Procd. Soc. Behv., 15, 3239–3243, https://doi.org/10.1016/j.sbspro.2011.04.278, 2011.
Clark, H. and Jones, J.: The use of a fieldwork audit to anticipate barriers to fieldwork for disabled students, Planet, 24, 42–49, https://doi.org/10.11120/plan.2011.00240042, 2011.
Cliffe, A. D.: A review of the benefits and drawbacks to virtual field guides in today's Geoscience higher education environment, International Journal of Educational Technology in Higher Education, 14, 1–14. https://doi.org/10.1186/s41239-017-0066-x, 2017.
Dolphin, G., Dutchak, A., Karchewski, B., and Cooper, J.: Virtual field experiences in introductory geology: Addressing a capacity problem, but finding a pedagogical one, Journal of Geoscience Education, 67, 114–130, https://doi.org/10.1080/10899995.2018.1547034, 2019.
Dunphy, A. and Spellman, G.: Geography fieldwork, fieldwork value and learning styles, International Research in Geographical and Environmental Education, 18, 19–28, https://doi.org/10.1080/10382040802591522, 2009.
Eide, C. H., Howell, J. A., and Buckley, S. J.: Sedimentology and reservoir properties of tabular and erosive offshore transition deposits in wave-dominated, shallow-marine strata: Book Cliffs, USA, Petrol. Geosci., 21, 55–73, https://doi.org/10.1144/petgeo2014-015, 2015.
Enge, H. D. and Howell, J. A.: Impact of deltaic clinothems on reservoir performance: Dynamic studies of reservoir analogs from the Ferron Sandstone Member and Panther Tongue, Utah, AAPG bulletin, 94, 139–161, https://doi.org/10.1306/07060908112, 2010.
Enge, H. D., Howell, J. A., and Buckley, S. J.: The geometry and internal architecture of stream mouth bars in the Panther Tongue and the Ferron Sandstone Members, Utah, USA, J. Sediment. Res., 80, 1018–1031, https://doi.org/10.2110/jsr.2010.088, 2010.
Esarey, J. and Valdes, N.: Unbiased, reliable, and valid student evaluations can still be unfair, Assess. Eval. High. Edu., 45, 1106–1120, https://doi.org/10.1080/02602938.2020.1724875, 2020.
Evelpidou, N., Karkani, A., Saitis, G., and Spyrou, E.: Virtual field trips as a tool for indirect geomorphological experience: a case study from the southeastern part of the Gulf of Corinth, Greece, Geosci. Commun., 4, 351–360, https://doi.org/5194/gc-4-351-2021, 2021.
Falivene, O., Arbués, P., Howell, J., Muñoz, J. A., Fernández, O., and Marzo, M.: Hierarchical geocellular facies modelling of a turbidite reservoir analogue from the Eocene of the Ainsa Basin, NE Spain, Mar. Petrol. Geol., 23, 679–701, https://doi.org/10.1016/j.marpetgeo.2006.05.004, 2006.
Fletcher, S., France, D., Moore, K., and Robinson, G.: Fieldwork education and technology: A GEES perspective, Planet, 7, 17–19, https://doi.org/10.11120/plan.2002.00070017, 2002.
Foxford, K. A., Walsh, J. J., Watterson, J., Garden, I. R., Guscott, S. C., and Burley, S. D.: Structure and content of the Moab Fault Zone, Utah, USA, and its implications for fault seal prediction, Geological Society, London, Special Publications, 147, 87–103, https://doi.org/10.1144/GSL.SP.1998.147.01.06, 1998.
Gazcón, N. F., Nagel, J. M. T., Bjerg, E. A., and Castro, S. M.: Fieldwork in Geosciences assisted by ARGeo: A mobile Augmented Reality system, Comput. Geosci., 121, 30–38, https://doi.org/10.1016/j.cageo.2018.09.004, 2018.
Giles, S., Jackson, C., and Stephen, N.: Barriers to fieldwork in undergraduate geoscience degrees, Nature Reviews Earth & Environment, 1, 77–78, https://doi.org/10.1038/s43017-020-0022-5, 2020.
Giorgis, S.: Google Earth mapping exercises for structural geology students – A promising intervention for improving penetrative visualization ability, Journal of Geoscience Education, 63, 140–146, https://doi.org/10.5408/13-108.1, 2015.
Google: Explore the World in 3D, https://earth.google.com/web/@13.33890973,-67.80216453, -2626.54264152a,23691900.2768d,35y,0h,0t,0r/data=CjkSNx IgZDY1OGRjYWIzNjlhMTFlOGFjNmU2OWJjN2I2ZDI2Y2 EiE2xheWVyXzNkY292ZXJfcGFuZWw last access: September 2021.
Gregory, D. D., Tomes, H. E., Panasiuk, S. L., and Andersen, A. J.: Building an online field course using digital and physical tools including VR field sites and virtual core logging, Journal of Geoscience Education, 70, 85–100, https://doi.org/10.1080/10899995.2021.1946361, 2022.
Harrald, J. E., Coe, A. L., Thomas, R. M., and Hoggett, M.: Use of drones to analyse sedimentary successions exposed in the foreshore, P. Geologist. Assoc., 132, 253–268, https://doi.org/10.1016/j.pgeola.2021.02.001, 2021.
Hartley, A. and Evenstar, L.: Fluvial architecture in actively deforming salt basins: Chinle Formation, Paradox Basin, Utah, Basin Res., 30, 48–166, https://doi.org/10.1111/bre.12247, 2018.
Hartley, A. J., Owen, A., Swan, A., Weissmann, G. S., Holzweber, B. I., Howell, J., Nichols, G., and Scuderi, L.: Recognition and importance of amalgamated sandy meander belts in the continental rock record, Geology, 43, 679–682, https://doi.org/10.1130/G36743.1, 2015.
Hesthammer, J., Fossen, H., Sautter, M., Sæther, B., and Johansen, S. E.: The use of information technology to enhance learning in geological field trips, Journal of Geoscience Education, 50, 528–538, https://doi.org/10.5408/1089-9995-50.5.528, 2002.
Horsman, E., Tikoff, B., and Morgan, S.: Emplacement-related fabric and multiple sheets in the Maiden Creek sill, Henry Mountains, Utah, USA, J. Struct. Geol., 27, 1426–1444, https://doi.org/10.1016/j.jsg.2005.03.003, 2005.
Howell J. and Flint S.: Sequence stratigraphy of the Book Cliffs Succession, in: The sedimentary record of sea-level change, edited by: Coe, A., Cambridge University Press, 9–40, ISBN 0-521-83111-3, 2003.
Howell, J. A., Martinius, A. W., and Good, T. R.: The application of outcrop analogues in geological modelling: A review, present status and future outlook, in: Sediment-Body Geometry and Heterogeneity: Analogue Studies for Modelling the Subsurface, Geological Society, London, Special Publication, 387, 1–25, https://doi.org/10.1144/SP387.12, 2014.
Howell, J. A. Eide, C. H. E., and Hartley, A. J.: No evidence for sea level fall in the Cretaceous strata of the Book Cliffs of Eastern Utah, EarthArXiv [preprint], https://doi.org/10.31223/osf.io/2ju3d, 2018.
Howell, J. A., Chmielewska, M., Lewis, C., Buckley, S., Naumann, N., and Pugsley, J.: Acquisition of Data for Building Photogrammetric Virtual Outcrop Models for the Geosciences using Remotely Piloted Vehicles (RPVs), EarthArXiv [preprint], https://doi.org/10.31223/X54914, 2021.
Hurst, S. D.: Use of “virtual” field trips in teaching introductory geology, Comput. Geosci., 24, 653–658, 1998.
Jackson, M. D., Hampson, G. J., and Sech, R. P.: Three-dimensional modeling of a shoreface-shelf parasequence reservoir analog: Part 2. Geologic controls on fluid flow and hydrocarbon recovery, AAPG Bull., 93, 1183–1208, https://doi.org/10.1306/05110908145, 2009.
Jacobson, A. R., Militello, R., and Baveye, P. C.: Development of computer-assisted virtual field trips to support multidisciplinary learning, Comput. Geosci., 52, 571–580, https://doi.org/10.1016/j.compedu.2008.11.007, 2009.
Kingsbury, C. G., Sibert, E. C., Killingback, Z., and Atchison, C. L.: “Nothing about us without us”: The perspectives of autistic geoscientists on inclusive instructional practices in geoscience education, Journal of Geoscience Education, 68, 302–310, https://doi.org/10.1080/10899995.2020.1768017, 2020.
Klippel, A., Zhao, J., Oprean, D. Wallfrün, J. O., Stubbs, C., La Famina, P., and Jackson, J. L.: The value of being there: toward a science of immersive virtual field trips, Virtual Reality, 24, 753–770, https://doi.org/10.1007/s10055-019-00418-5, 2020.
Kuorikoski, J. and Ylikoski, P.: External representations and scientific understanding, Synthese, 192, 3817–3837, https://doi.org/10.1007/s11229-014-0591-2, 2015.
Lisle, R. J.: Google Earth: a new geological resource, Geology Today, 22, 29–32, https://doi.org/10.1111/j.1365-2451.2006.00546.x, 2006.
Litherland, K. and Stott, T. A.: Virtual field sites: Losses and gains in authenticity with semantic technologies, Technol. Pedagog. Educ., 21, 213–230, https://doi.org/10.1080/1475939X.2012.697773, 2012.
Lukes, L.: A new take on the field trip: A low-tech, inquiry-based virtual field experience, The Science Teacher, 8, 24–29, 2014.
Matthews, W., Hampson, G., Trudgill, B., Underhill, J. and Post, P. J.: Impact of salt movement on fluvio-lacustrine stratigraphy and facies architecture: Late Triassic Chinle Formation, northern Paradox Basin, SE Utah, USA, in: Salt-sediment interactions and hydrocarbon prospectivity: Proceedings of 24th Annual Gulf Coast Section SEPM Foundation Bob F. Perkins Research Conference, Houston, TX, 931–964, 2004.
Mogk, D. W. and Goodwin, C.: Learning in the field: Synthesis of research on thinking and learning in the geosciences, in: Earth and Mind II: A Synthesis of Research on Thinking and Learning in the Geosciences, edited by: Kastens, K. A. and Manduca, C. A., Geological Society of America, 2, 131–164, https://doi.org/10.1130/2012.2486(24), 2012.
Monet, J. and Greene, T.: Using Google Earth and satellite imagery to foster place-based teaching in an introductory physical Geology course, Journal of Geoscience Education, 60, 10–20, https://doi.org/10.5408/10-203.1, 2012.
Nichols, G. J.: The structure and stratigraphy of the western external sierras of the Pyrenees, northern Spain, Geol. J., 22, 245–259, https://doi.org/10.1002/gj.3350220307, 1987.
Nichols, G. J. and Hirst, J. P.: Alluvial fans and fluvial distributary systems, Oligo-Miocene, northern Spain; contrasting processes and products, J. Sediment. Res., 68, 879–889, https://doi.org/10.2110/jsr.68.879, 1998.
Owen, A., Nichols, G. J., Hartley, A. J., Weissmann, G. S., and Scuderi, L. A.: Quantification of a distributive fluvial system: the salt wash DFS of the Morrison Formation, SW U.S.A., J. Sediment. Res., 85, 544–561, https://doi.org/10.2110/jsr.2015.35, 2015.
Pattison, S. A.: Sequence stratigraphic significance of sharp-based lowstand shoreface deposits, Kenilworth Member, Book Cliffs, Utah, AAPG bulletin, 79, 444–462, https://doi.org/10.1306/8D2B155C-171E-11D7-8645000102C1865D, 1995.
Peat, M. and Taylor, C.: Virtual biology: How well can it replace authentic activities, CAL-Laborate, 13, 21–24, 2005.
Petersen, G. B., Klingenberg, S., Mayer, R. E., and Makransky, G., The virtual field trip: Investigating how to optimize immersive virtual learning in climate change education, Brit. J. Educ. Technol., 51, 2099–2115, https://doi.org/10.1111/bjet.12991, 2020.
Phillips, S. P., Howell, J. A., Hartley, A. J., and Chmielewska, M.: Tidal estuarine deposits of the transgressive Naturita Formation (Dakota Sandstone): San Rafael Swell, Utah, USA, J. Sediment. Res., 90, 777–795, https://doi.org/10.2110/jsr.2020.51, 2020.
Phillips, S. P., Howell, J. A., Hartley, A. J., Chmielewska, M., and Hudson, S. M.: Evolution of foreland basin fluvial systems in the mid-Cretaceous of Utah, USA (upper Cedar Mountain and Naturita formations), Sedimentology, 5, 2097–2124, https://doi.org/10.1111/sed.12845, 2021.
Pickering, K. T. and Corregidor, J.: Mass-transport complexes (MTCs) and tectonic control on basin-floor submarine fans, middle Eocene, south Spanish Pyrenees, J. Sediment. Res., 75, 761–783, https://doi.org/10.2110/jsr.2005.062, 2005.
Pringle, J. K., Howell, J. A., Hodgetts, D., Westerman, A. R., and Hodgson, D. M.: Virtual outcrop models of petroleum reservoir analogues: a review of the current state-of-the-art, First Break, 24, 33–42, https://doi.org/10.3997/1365-2397.2006005, 2006.
Ramasundaram, V., Grunwald, S., Mangeot, A., Comerford, N. B., and Bliss, C.: Development of an environmental virtual field laboratory, Comput. Educ., 45, 21–34, https://doi.org/10.1016/j.compedu.2004.03.002, 2005.
Rittersbacher, A., Buckley, S. J., Howell, J. A., Hampson, G. J., and Vallet, J.: Helicopter-based laser scanning: a method for quantitative analysis of large-scale sedimentary architecture, Geological Society, London, Special Publications, 387, 185–202, https://doi.org/10.1144/SP387.3, 2015.
Rotevatn, A., Tveranger, J., Howell, J. A., and Fossen, H.: Dynamic investigation of the effect of a relay ramp on simulated fluid flow: geocellular modelling of the Delicate Arch Ramp, Utah, Petrol. Geosci., 15, 45–58, https://doi.org/10.1144/1354-079309-779, 2009.
Rotzien, J. R., Sincavage, R., Pellowski, C., Gavillot, Y., Filkorn, H., Cooper, S., Shannon, J., Yildiz, U., Sawyer, F., and Uzunlar, N.: Field-Based Geoscience Education during the COVID-19 Pandemic: Planning, Execution, Outcomes, and Forecasts, GSA Today, 31, 4–10, https://doi.org/10.1130/GSATG483A.1, 2021.
Safaridb: https://safaridb.com/, last access: 4 September 2021.
Schott, C.: Virtual fieldtrips and climate change education for tourism students, J. Hosp. Leis. Sport To., 21, 13–22, https://doi.org/10.1016/j.jhlste.2017.05.002, 2017.
Sømme, T. O., Howell, J. H., and Hampson, G. J.: Architecture and genesis of intra-parasequence discontinuity surfaces in wave-dominated deltaic deposits: Upper Cretaceous Sunnyside Member, Book Cliffs, Utah, U.S.A., in: Recent Advances in Models of Siliciclastic Shallow-Marine Stratigraphy, edited by: Hampson, G. J., Steel, R. J., Burgess, P. M., and Dalrymple, R. W., SEPM (Society for Sedimentary Geology) Special Publication, 90, 437–456, 2008.
Spooren, P., Brockx, B., and Mortelmans, D.: On the Validity of Student Evaluation of Teaching: The State of the Art, Rev. Educ. Res., 83, 598–642, https://doi.org/10.3102/0034654313496870, 2013.
Stainfield, J., Fisher, P., Ford, B., and Solem, M.: International virtual field trips: a new direction?, J. Geogr. Higher Educ., 24, 255–262, https://doi.org/10.1080/713677387, 2000.
Stumpf II, R. J., Douglass, J., and Dorn, R. I.: Learning desert geomorphology virtually versus in the field, J. Geogr. Higher Educ., 32, 378–399, https://doi.org/10.1080/03098260802221140, 2008.
Tibaldi, A., Bonali, F. L., Vitello, F., Delage, E., Nomikou, P., Antoniou, V., Becciani, U., de Vries, B. V. W., Krokos, M., and Whitworth, M.: Real world–based immersive Virtual Reality for research, teaching and communication in volcanology, B. Volcanol., 82, 1–12, https://doi.org/10.1007/s00445-020-01376-6, 2020.
V3Geo: https://v3geo.com, last access: 4 September 2021.
Van Wagoner, J. C.: Sequence Stratigraphy and Marine to Nonmarine Facies Architecture of Foreland Basin Strata, Book Cliffs, Utah, AAPG, 64, 9–21, https://doi.org/10.1306/M64594, 1995.
Whitmeyer, S. J. and Dordevic, M.: Creating virtual geologic mapping exercises in a changing world, Geosphere, 17, 226–243, https://doi.org/10.1130/GES02308.1, 2021.
Whitmeyer, S. J., Feely, M., De Paor, D. G., Hennessy, R., Whitmeyer, S., Nicoletti, J., Santangelo, B., Daniels, J., and Rivera, M.: Visualization techniques in field geology education: A case study from Western Ireland, 2009, in: Field Geology Education: Historical Perspectives and Modern Approaches, edited by: Whitmeyer, S. J., Mogk, D. W., and Pyle, E. J., Geological Society of America Special Paper, 461, 105–115, 2009.
Yu, L. and Gong, P.: Google Earth as a virtual globe tool for Earth science applications at the global scale: progress and perspectives, Int. J. Remote Sens., 33, 3966–3986, https://doi.org/10.1080/01431161.2011.636081, 2011.
Xu, X., Aiken, C. L., Bhattacharya, J. P., Corbeanu, R. M., Nielsen, K. C., McMechan, G. A., and Abdelsalam, M. G.: Creating virtual 3-D outcrop, The Leading Edge, 19, 197–202, https://doi.org/10.1190/1.1438576, 2000.
- Abstract
- Introduction
- Previous work on VFTs
- Learning objectives and planning
- Field trip software and applications overview
- Field trip design and delivery
- Methodology for evaluation
- Evaluation results
- Discussion and conclusions
- Conclusions
- Data availability
- Author contributions
- Competing interests
- Disclaimer
- Special issue statement
- Acknowledgements
- Review statement
- References
- Supplement
- Abstract
- Introduction
- Previous work on VFTs
- Learning objectives and planning
- Field trip software and applications overview
- Field trip design and delivery
- Methodology for evaluation
- Evaluation results
- Discussion and conclusions
- Conclusions
- Data availability
- Author contributions
- Competing interests
- Disclaimer
- Special issue statement
- Acknowledgements
- Review statement
- References
- Supplement