the Creative Commons Attribution 4.0 License.
the Creative Commons Attribution 4.0 License.
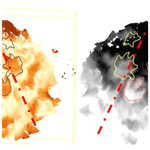
Introduction to teaching science with three-dimensional images of dinosaur footprints from Cristo Rey, New Mexico
Valeria V. Martinez
Laura F. Serpa
In this paper we discuss the use of three-dimensional (3-D) imagery and virtual field trips to teach pre-university and non-major university geoscience courses. In particular, 3-D PDF (Portable Document Format) files can be used to either prepare students for or completely replace a field trip when logistical problems make the actual trip too difficult to be effective or when some students need an alternative accommodation. Three-dimensional images can replace or supplement classroom activities, such as the identification of rocks and minerals from hand samples or the identification of geologic structures from 2-D photographs and limited field observations. Students can also become involved in data collection and processing to further their understanding of photogrammetry and visualization. The use of 3-D imagery can make additional time available to instructors to cover more advanced topics and teach students more about the role of science in geologic research.
We use an example from Cristo Rey, New Mexico, where dinosaur footprints and tracks are present but difficult to see in many cases, and they are often in places that are hard to access for many people. At this site, approximately 10 000 photographs were collected and processed as 3-D images to show one approximately 72 m2 area of known footprints. However, we also conducted some very simple digital manipulations of the images that allowed us to identify new footprints and tracks that were not apparent when viewed in the field. The photographs and 3-D images have been donated to the Insights El Paso Science Center (denoted Insights Museum herein) that owns the fossil site, and they are now being used to develop educational materials and lessons for the nearby communities.
- Article
(10293 KB) - Full-text XML
-
Supplement
(18103 KB) - BibTeX
- EndNote
The current pandemic has changed education in many ways, but few areas of science education have been impacted as much as the geological sciences where field observations and trips are a significant part of most geoscience curricula. During the pandemic, many universities replaced field trips and exercises with a variety of virtual field experiences (Rotzien et al., 2021) despite some prior faculty reticence to the use of computer-based tools for field mapping classes and other field activities. In addition, prior to the pandemic there was a growing trend at many universities and schools in the USA to eliminate or restrict field activities because of administrative concerns about student liability, cost, and, in some cases, the lack of faculty able and/or willing to teach field-based classes on a regular basis. It is our opinion that the pandemic will be a major factor in a permanent decrease in the number of field-based geology activities in US universities. Furthermore, we expect that field observational geology will become virtual for nearly all pre- or early-college students who are not majoring in a geoscience.
One critical question regarding the changing emphasis on field experiences (Whitmeyer et al., 2020) in geoscience education is whether field-based activities are truly essential and where might they be most valuable. The authors of this paper are experienced geoscientists with considerable field experience, but we contend that there are some significant benefits to the use of technology in geoscience education. We argue that non-majors at universities and students in the pre-university system may learn more from virtual field activities than they might learn from actual field-based observations. In particular, we see that the use of three- and four-dimensional photogrammetry models and videos, as well as many other developing technologies like artificial intelligence and large-scale statistical methods (Whitmeyer et al., 2019), has an educational advantage over field work, because these methods of delivery are more relevant to many students. In addition, the incorporation of virtual activities and lessons in a modern textbook should provide more time for discussions of what geoscience researchers actually do and why that is important science. To test this concept, we are working with a local museum to develop educational materials on dinosaur footprints, and that effort is described below.
1.1 Study area
Cristo Rey, New Mexico (Fig. 1), is the site of numerous Cretaceous dinosaur footprints and trackways that are particularly difficult to recognize and map (Kappus and Cornell, 2003; Kappus et al., 2011). Weathering patterns obscure or mimic many of the fossils and the locality experienced Tertiary uplift and tilting that deformed the site and made the terrain difficult to navigate. The fossil site is located adjacent to the US–Mexico border near the cities of Las Cruces, NM, and El Paso, TX, and this makes it a potentially valuable local education resource. The donation of the land to the Insights Museum in 2011 opened the site to educational groups and the development of exhibits, but that work is still in early development. In an effort to preserve as much information as possible on the dinosaur footprints and to provide students and teachers with a non-invasive way to study the fossils, Martinez (2016) created a detailed high-resolution photographic record of a portion of the site and used that data to build 3-D images of the ground surface. Those images not only provide a record of the site but also led to the identification of several new footprints and fossils based on digital manipulation of the images.
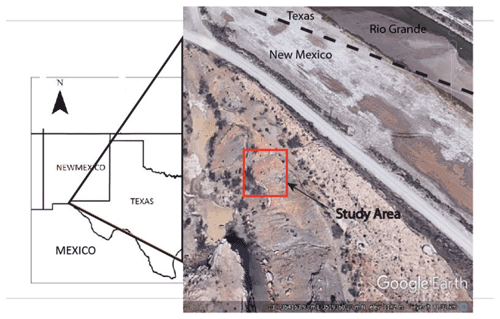
Figure 1Map of the region modified from ©Google Earth (2021). The red box in the image shows the study area in Cristo Rey, New Mexico.
The use of 3-D models to study fossils is a relatively recent technique (Djuricic et al., 2016; Bates et al., 2008; Adams et al., 2010; Remondino et al., 2010; Tavani et al., 2014) that has advantages over methods such as paper mapping, plaster casts or collecting samples as it is non-destructive and can be easily shared with a wide audience. Our preliminary study of the images we collected (Martinez, 2016) shows numerous Cretaceous Theropoda, Iguanodon, and Ankylosaurus footprints (Fig. 2) previously identified by Kappus et al. (2010) and Kappus and Cornell (2003) as well as new footprints and trails of those dinosaurs that were not previously recognized. These images were donated to the Insights Museum and we are helping to develop educational resources for the local community to encourage studies of the fossils.
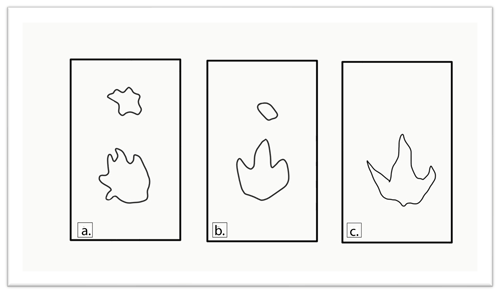
Figure 2The general shapes of the three types of Cretaceous dinosaur footprints identified (Kappus and Cornell, 2003; Kappus et al., 2011; Martinez, 2016) at Cristo Rey. Panel (a) is an ankylosaur, panel (b) is an iguanodon, and panel (c) is a theropod (outlines modified from Kappus et al., 2011).
Our educational activities focus on both methodology and fossil identification for several age groups. In the USA, geology is often taught in middle schools for 11- to 13-year-old students. It is also a popular choice for university students who do not want to use mathematics but are required to take at least one science course. We are focusing on the use of 3-D images that can be easily used in the classroom and manipulated by students with minimal expense or expertise. Our intent is to use this approach to increase interest in the study of geology amongst these groups of students, so we can increase the rigor of our courses over time. Initially the fossil identification activities will follow traditional lessons plans that include the identification of dinosaurs based on their footprints, estimating the size and speed of a dinosaur based on the measured stride (Thulborn, 1990), and looking at dinosaur activities in relationship to their environmental setting (Lockley, 2011, 1986). We anticipate that the Insights Museum will expand on our preliminary work, but we will work closely with them to develop some initial lessons based on our own experience of discovery at the site.
1.2 Geologic background
The first fossil footprints, identified as Cretaceous theropod footprints, were discovered by Kappus and Cornell (2003). The footprints are in Cretaceous sedimentary deposits that were intruded by a Tertiary trachyandesite dome (Lovejoy, 1976). The dinosaur tracks are located primarily in the upper sandstone of the Anapra formation, which is a massive cross-bedded arkosic sandstone varying in thickness from 1–2 m and interbedded with grey and purple shale (Lovejoy, 1976). These sedimentary layers indicate that the Cretaceous environment in this area was a shallow sea (Kappus et al., 2011).
1.3 Methods
Most of the initial research on the Cristo Rey tracks consisted of field notes, photos, and drawings (Kappus and Cornell, 2003; Kappus et al., 2011). More recently, technology has become available to create 3-D visualizations of geologic units (Carrivick et al., 2016), and this is changing the way fossils are studied (Remondino et al., 2010). Eric Kappus, Jose Hurtado, and Raed AlDouri (personal communication, 2016) used lidar to record the Cristo Rey footprints to produce the first 3-D images of the site. The resulting anaglyph 3-D images require anaglyph glasses to see and cannot be easily manipulated on a computer, but they provide the inspiration for continued work in this area.
1.4 Data collection
The original goal of this project was to create a digital record that could be used in educational programs and research that did not require field visits in all cases. The materials needed for this project can be easily obtained and used with teachers and their students (Fleming and Pavlis, 2018). However, we did use materials in this project that may not be readily available to most teachers. For example, we used a Nikon camera series D3100 digital single-lens reflex (DSLR) camera for our photography and Agisoft Photoscan Pro™ and ENVI™ software for processing and data analysis, which may not be affordable for most teachers. However, a cell phone camera and several inexpensive and free software packages can be used if the data set is kept small (Fleming and Pavlis, 2018).
We collected approximately 10 000 photographs and reduced the number to 7600 photographs using a grid system that was approximately 9 m across in the x direction and 8 m across in the y direction (Fig. 3). Four people were needed for the data collection with one located at position (0,0) to establish the origin position, one person measuring the x coordinates with a measuring tape, another measuring the y coordinates with a second measuring tape (Fig. 4), and one using a meterstick to precisely mark grid points on the outcrop using temporary adhesive stickers labeled with the point's coordinate number (Fig. 5). The lead author, Martinez, developed the grid system, supervised the grid measuring process, and took all the photographs. At each grid intersection, a suite of photographs was taken at a distance of ∼ 1 m from the surface with the camera directed into the adjacent grid areas. This was done at each grid position until all four corners had been occupied for photography. The total number of pictures taken at each grid position depended on the surface texture and variations in the surface of the target. For example, if there were a lot of variations in the target surface, then more pictures were taken in order to capture sufficient details for subsequent modeling.
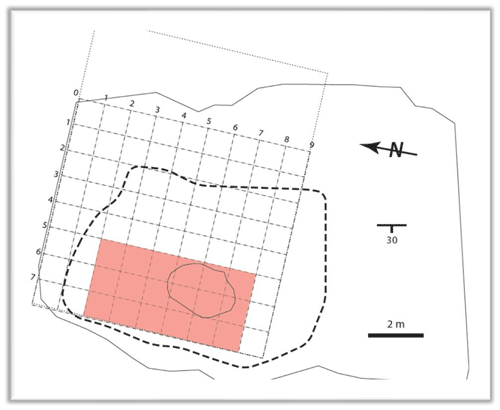
Figure 3Grid layout used to take photographs. The grid spacing is 1 m, and photos were taken from each grid intersection with a camera aimed into the target grid space. The shaded area shows the area we processed for this study, and the heavy dashed line outlines the map area from Kappus et al. (2011).
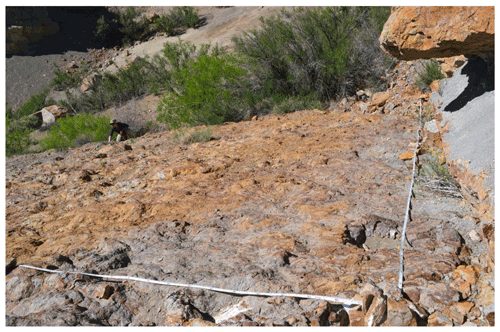
Figure 4The grid system used in this study was based on two metric tape measures laid out perpendicular to each other to establish the locations for photographs. The positions of the individual grid intersections at 1 m spacing were marked as shown in Fig. 5.
1.5 Data processing
The images were processed using the structure from motion (SfM) photogrammetry technique (Westoby et al., 2012). The photogrammetric processing was done using Agisoft Photoscan Pro™ software to create a 3-D model from the photos. Each set of photos for a single grid section was processed individually to form a 1 m × 1 m “chunk” (Agisoft, 2021), because the data set was too large to process the entire set as a single unit. Once all the chunks were created, they were arranged to form larger chunks, starting with grid positions (0,0) and (0,1). In this fashion, the chunks were iteratively aligned and merged to make progressively bigger chunks.
In 2015–2016 we were limited by available computing power and did not have sufficient RAM and CPU power to create a single model for the entire outcrop. For that reason, we chose to work on a subset of our data in an area where Kappus et al. (2011) found the most dinosaur footprints (Fig. 3). In early 2021 we used Pix4D™ software to reprocess some of the data for this paper and have had some success, but we have not yet been able to produce a single model of the entire area. There is insufficient overlap of photos between some of the grid areas to use the SfM software to completely merge all of the images into a single model (Wenzel et al., 2013). The method we used to collect data focused on coverage within each grid area but not on the connectivity between grid areas.
Besides the problems with the lack of overlap between grid sections in many of our photos, the camera was also too close to the surface to accurately capture all of the topographic relief in the region (James et al., 2017). We also did not have position information for the individual photos, so it was not possible to georeference the data (Brush et al., 2019). This was our first effort to collect photogrammetry data, and we recognize our mistakes. We subsequently collected small unmanned aerial vehicle (sUAV, i.e., drone) and lidar data which we will compare to our hand-held photography in the near future. Despite the problems with the initial work, we have high-quality photos of the entire fossil site and can produce 3-D images of the individual gridded sections independently or in small groups. This provides us with an excellent educational data set that students and teachers can access freely and learn more about fossils and photogrammetry techniques.
As noted above, our photos were not georeferenced and lacked sufficient overlap to produce a continuous 3-D model of the area. When we collected the data in 2015, we had no previous experience with photogrammetry and only knew that a lot of photos from a range of orientations were needed to produce a model. This experience is one of several early efforts (Brush et al., 2019; Pavlis and Mason, 2017; Fleming and Pavlis, 2018) among a group of faculty and students in our department to explore photogrammetry as a potential tool for geoscience research and education. We have subsequently learned a great deal more about photogrammetry, and we recognize the need to pre-plan a project with careful consideration of goals and methodology (Brush et al., 2019; James et al., 2017; Carrivick et al., 2016; Bemis et al., 2014; Wenzel et al., 2013).
The photographs described in this paper make up our initial donation to the Insights Museum to provide a permanent record of the fossil site. In addition, we will provide 3-D images made with the SfM software (cloud data) that can be distributed in a variety of formats. The basic format for the cloud data as seen in Figs. 6 and 7 is LAS (LASer), a format commonly used for lidar point cloud data, which can be manipulated using software like Cloud Compare™, which is freeware and has the ability to make depth measurements and comparisons with other data sources and many other relevant applications. In addition, we can post images on the web and produce 3-D PDF files (see the Supplement) that can be opened using software from Adobe™ on most standard laptop computers and tablets. For this project, we are focusing on the 3-D PDF images, because most schools and universities have access to these files and the Adobe Acrobat software necessary to interact with them. In many US schools, students are not allowed to access some websites, and in particular, Sketchfab is usually not considered appropriate for pre-college-age students although it is an excellent site for posting 3-D images. Adobe Acrobat has several options for manipulating the 3-D PDF files to help students recognize fossils and they can be accessed without a direct connection to the internet.
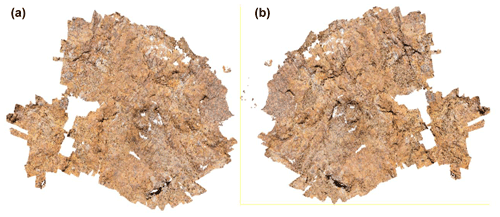
Figure 6Front and back view of Cristo Rey 3-D PDF image from grid sections (3, 4) to (3, 5) and (4, 4) to (4, 6). Each image occupies an area of approximately 2 m by 2 m. Panel (a) shows what a field observer would see in the fossil locality, and panel (b) shows the same image as panel (a) but reversed to show the back side of the fossil image which a field observer would not see.
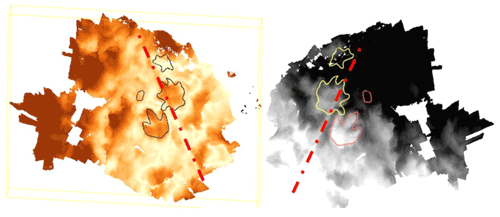
Figure 7The same images shown in Fig. 6 but the natural color of the images has been replaced by colors that vary with depth. The dashed red line shows the location of a fracture in the rocks, and the footprints are outlined in this image.
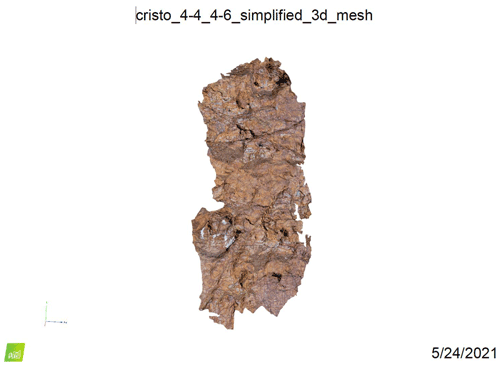
Figure 8This figure is a 2-D image of the 3-D PDF of Cristo Rey sections 4–5 and 4–6 included in the Supplement to this paper.
The original fossils identified by Martinez (2016) were from a small set of photos where she removed the color from a 3-D PDF image and found that the weathering patterns in the rocks obscured the footprints and distracted from recognition of the fossil relief. This was first observed by simply turning the 3-D PDF over and looking at the back side which had no color at that time and showed the footprint as a high area rising above the background rock. Subsequent mapping of depth variations at a small scale combined with color removal made more footprints and tracks apparent in the site as shown in Figs. 6 and 7.
2.1 Fossil identification
Our experience suggests that most students first learn about fossils by seeing a few examples, usually photographs in a textbook, and learning to recognize some basic characteristics of a fossil that can be used to identify the source. For students living near a fossil locality, this may also involve a field trip to collect fossils. However, some fossil localities are rapidly disappearing as the fossils have all been collected. Cristo Rey fossils have been heavily collected and most remaining fossils are in hard to access areas that may not be safe to visit for young people with little or no field experience. We also hope that the remaining fossils will not be collected so that scientific studies of the footprints can be conducted well into the future.
Figures 6 and 7 show images from a part of the area that includes two footprints and are photographs of the actual 3-D dense cloud models. A 3-D PDF of that area is included in the Supplement, and a 2-D image of the figure is shown in Fig. 8. The supplemental 3-D PDF file should be manipulatable with Adobe Acrobat Pro™ with 3-D enabled but may take a few minutes to open. Readers and students can change the orientation and tilt of the 3-D image to bring out varying views of the depth and highlight the mud ridges around the footprint. The image on the left side of Fig. 6 shows a view of what would be seen in the field if the lighting were good. However, because the students will have a 3-D image (Fig. 8) to look at, they will be able to manipulate it with a computer, tablet, or smart phone in ways that are not available to the field observer. One of the first things we did with the images when we started this project was to turn the 3-D PDF image over and look at the back side where footprints that are depressed in the top side pop out as raised impressions on the back side of the image as shown on the right side of Fig. 6.
The footprints may be difficult for students to find initially because there is a lot of color variations in the rocks. However, we can change the colors in the computer image also; Fig. 7. shows the same images as shown in Fig. 6 but the true colors are replaced by two tone color variations that change intensity with depth. In this format, the most visible footprint is easily seen as a depressed area that is darker brown than the surrounding, yellow high areas in the right-hand image and as a light area in the backside of the image where we used grey tones. Students can experiment with their image colors, orientation or scale to find a representation that helps them to visualize the footprints and learn something about how visualization works in the process.
Once students learn to find the footprints in the image, they can compare them to published information (Thulborn, 1990) to identify the dinosaurs that made the prints. Students can also measure the prints and if there are sufficient prints, they can measure the stride of the dinosaurs to make an estimate of the sizes of the dinosaurs. Some additional lessons that can be included might be to look at how mud was apparently pushed away from the footprint and compare that to how a human footprint changes when a person runs compared to a walking person's footprint in mud. In some cases, students might consider how a footprint would look if the dinosaur were pushing along in shallow mud while swimming. This would provide additional information about the environment and lifestyle of the dinosaurs (Boggs, 2011).
This data can also give teachers a chance to develop their students' critical thinking skills. Cristo Rey is located in a desert region, but the dinosaurs clearly lived in a shallow water environment in our area which could open a discussion on climate change (Dalla Vecchia, 2008), for example. Comparing how the students might displace mud in a wet environment and observing similar features in the dinosaur footprints could create a better understanding of how science works and builds understanding of the processes we study. The mud ridges around the footprints also provide evidence the dinosaurs were walking on a sloping surface which was subsequently tilted in the opposite direction during uplift of the area. In addition, some footprints overlap other footprints which opens discussions of the time relationships and whether one of the types of dinosaurs found in our area could have lived there at a much earlier time than another. These observations would make an excellent exercise for the students to explore with the entire data set.
2.2 Photogrammetry
In addition to learning about fossils, students can learn more about the process of visualization and making 3-D images from the more than 7000 photos available from the Insights Museum. There are inexpensive or free software packages available to work with smartphone images to build 3-D models (Fleming and Pavlis, 2018). This gives students an excellent opportunity to learn more about how humans perceive depth and dimensionality, for example. Where more sophisticated software is available, students can work on building a geologically accurate representation of the Cristo Rey fossil sites. This should also give students a good understanding and the necessary skills to look at rocks and minerals, for example, or any number of subjects in 3-D which is likely to be common in their future regardless of the career they choose.
The methods used in this project are not intended for rigorous research projects where accurate measurements are critical. Rather, we are focusing on the visualization aspect of photogrammetry and making it easily accessible to teachers and students in a wide range of applications. For more advanced courses and research applications, it will be critical to train students in the details of properly planning a project (James et al., 2017; Wenzel et al., 2013; Brush et al., 2019; Fleming and Pavlis, 2018) to ensure they get the intended information from their photos. We also hope that properly collected research data will become available for education applications. However, more casual application of photogrammetry in educational settings is an effective method to build interest in a topic and, more importantly, to expand the level of information available in a classroom or textbook from 2-D images to 3- and even 4-D images.
At many US universities, introductory geology is taught using a small set of rock and mineral samples in a lab often combined with field excursions that may be taught by graduate students with little training in the broad range of topics covered in the lab. Introductory physical geology textbooks and lab manuals typically devote 4 to 5 chapters to rock and mineral identification and a chapter on, for example, structural geology that uses photographs or sketches where a student is expected to memorize the names of the features in the image. One reason for this is that geoscience is often not taught at the high school level (ages 14 to 18 typically) and there are relatively few geoscience majors entering the universities as freshmen. As a result, departments try to recruit majors from the students who choose to take a geology course to meet a science elective or because their major requires it. Those students are often not well prepared for a more rigorous science course and departments may focus on making their course interesting, informative, and relevant but usually not as difficult as, for example, an introductory physics or chemistry course. We compare this to students taking an introductory university biology class that is based almost entirely on pictures, dissecting animals, and field trips to collect plants and insects. Many students might enjoy such a class, but it is not likely to inspire many of them to become research scientists when this method is used at the university level.
We are not suggesting that field experiences or working in a lab with samples is not valuable, particularly in more advanced classes, but many universities train teachers who only take one introductory geology class before they teach a class in the pre-college system. As a result, few students learn much about what makes geology a science or arrive at universities with the intent of becoming a geologist. We believe the increasing availability of 3-D images that can show a wide range of scales and include videos on a tablet or cell phone frees up an instructor and textbook to explain the processes behind the images and how a geologist studies the Earth using sophisticated equipment in greater detail than is possible with a 2-D image. We also have the flexibility of linking specific images and lessons from Microsoft Word documents to PowerPoint lessons to aid students in viewing the lesson digitally.
The use of 3-D PDF files in a classroom or textbook is just the first of many possible uses of digital data and software to give students a sample of the tools that many researchers use regularly. The tools that we used to generate the 3-D PDF files from the photographs can easily be accessed and manipulated on a smartphone or tablet. The same methods can be applied to rocks and minerals, outcrops, and large-scale geologic features. Rotzien et al. (2021) used 3-D Google Earth images to prepare students for field mapping. Google Earth is often used in labs and introductory classes and textbooks so this is not a new idea. However, Google Earth is not usually of high enough resolution to see the detailed outcrop images or identify rock types or fossils. It cannot be manipulated with other software, so it is not the ideal tool for the lessons we think students should have. We believe the primary outcome of using higher-level technology in introductory classes is that students will see geoscience more as a rigorous science than as something that should be left to pre-university level courses with teachers who may not see the subject as a “real” or quantitative science.
We would like to see a change in how introductory university geoscience is taught with more emphasis on understanding the nature and process of science and its applications through the use of a broad range of high-resolution digital imagery. Exercises could involve looking at a variety of characteristics from one area or seeing the same geologic feature in different places and varying stages of development, for example. Every university could develop virtual field trip activities using digital data from their local area, so students can learn about why geology is relevant to their lives. Software, such as StraboSpot (https://Strabospot.org, last access: April 2018; Walker et al., 2019), is becoming more available and could provide the tools for rewriting our non-majors' textbooks, so students get a more realistic idea of how the Earth works and what a geoscientist does. The possibilities for developing virtual class materials are growing rapidly, and we think now is the time to bring it into the classroom.
The photographs and images used in this work are currently at the Insights Museum (http://www.insightselpaso.org/, last access: 27 November 2021) but are not yet available at the time of this writing.
The supplement related to this article is available online at: https://doi.org/10.5194/gc-5-1-2022-supplement.
The data were collected, processed, and analyzed by VVM for her master's thesis under the supervision of LFS. LFS subsequently reprocessed some of the data and assisted in the preparation of this article.
The contact author has declared that neither they nor their co-author has any competing interests.
Publisher’s note: Copernicus Publications remains neutral with regard to jurisdictional claims in published maps and institutional affiliations.
This article is part of the special issue “Virtual geoscience education resources”. It is not associated with a conference.
The authors greatly appreciate the assistance of Eric Kappus in identifying the fossils recorded in the data. The reviewers, Lisa White and an anonymous reviewer, provided valuable improvements to the text. We particularly thank the many students and faculty at the University of Texas at El Paso who assisted in the data collection and early learning experiences working with photogrammetry.
This paper was edited by Simon Buckley and reviewed by Lisa D. White and one anonymous referee.
Adams, T. L., Stranac, C., Polcyn, M. J., and Jacobs, L. L.: High Resolution Three Dimensional Laser-scanning of the Type Specimen of EubronetsGlenrosenis Shuler, 1935, from the Comanchean (Lower Cretaceous) of Texas: Implications for Digital Archiving and Preservations, Palaentologia Electronica, 13, 1T, 11 pp., available at: http://palaeo-electronica.org/2010_3/226/index.html (last access: 27 November 2021), 2010.
Agisoft: Agisoft PhotoScan User Manual: Professional Edition, Version 1.2, available at: http://www.agisoft.com/pdf/photoscan-pro_1_2_en.pdf, last access: 27 November 2021.
Bates, K. T., Manning, P. L., and Hodgetts, D.:Three-Dimensional Modelling and Analysis of Dinosaur Tracks, Paleontology, 51, 999–1010, https://doi.org/10.1111/j.1475-4983.2008.00789.x, 2008.
Bemis, S., Micklethwaite, S., Turner, D., James, M., Akciz, S., Thiele, S., and Bangash, H.: Ground-based and UAV-Based photogrammetry: A multi-scale, high-resolution mapping tool for structural geology and paleoseismology, J. Struct. Geol., 69, 163–178, https://doi.org/10.1016/j.jsg.2014.10.007, 2014.
Boggs Jr., S.: Principles of Sedimentology and Stratigraphy, 5th Edn., Pearson, Upper Saddle River, New Jersey, USA, 2011.
Brush, J., Pavlis, T., Hurtado, J., Mason, K., Knott, J., and Williams, K.: Evaluation of field methods for 3-D mapping and 3-D visualization of complex metamorphic structure using multiview stereo terrain models from ground-based photography, Geosphere, 15, 188–221, https://doi.org/10.1130/GES01691.1, 2019.
Carrivick, J., Smith, M., and Quincey, D.: Structure from Motion in the Geosciences, John Wiley and Sons, West Sussex, UK, https://doi.org/10.1002/9781118895818, 2016.
Dalla Vecchia, F. M.: The impact of dinosaur palaeoichnology in palaeoenvironmental and palaeogeographic reconstrucions: The case of the Periadriatic carbonate Platform, Oryctos, 8, 89–106, 2008.
Djuricic, A., Dorninger, P., Nothegger, C., Harzhauser, M., Szekely, B., Rasztovits, S., Mandic, O., Molnar, G., and Pfeifer, N.: High-resolution 3D surface modeling of a fossil oyster reef, Geosphere, 12, 1457–1477, https://doi.org/10.1130/GES01282.1, 2016.
Fleming, Z. and Pavlis, T.: An orientation based correction method for SfM-MVS point clouds–Implications for field geology. J. Struct. Geol., 76–89, https://doi.org/10.1016/j.jsg.2018.05.014, 2018.
James, M., Robson, S., and Smith, M.: 3-D uncertainty-based topographic change detection with structure-from-motion photogrammetry: precision maps for ground control and directly georeferenced surveys, Earth Surface Processes and Landforms, 42, 1769–1788, https://doi.org/10.1002/esp.4125, 2017.
Kappus, E. and Cornell, W. C.: A New Cretaceous Dinosaur Tracksite in Southern New Mexico, Palaentologia Electronica, 6, 6 pp., available at: https://palaeo-electronica.org/2003_1/track/issue1_03.htm (last access: 27 November 2021), 2003.
Kappus, E., Lucas, S., Hunt, A., Heckert, A., and Lockley, M.: Dinosaur Footprints from the Lower Cretaceous Sarten Member of the Mojado Formation at Cerro de Cristo Rey, Dona Ana County, New Mexico, Ichnos, 10, 263–267, https://doi.org/10.1080/10420940390257905, 2010.
Kappus, E. J., Lucas, S. G., and Langford, R.: Cerro de Cristo Rey Cretaceous Dionsar Tracksites, Sunlad Park, New Mexico, USA, and Chihuahua, Mexico, New Mexico Museum of Natural History and Science, 272–288, 2011.
Lockley, M. G.: The Paleobiological and Paleoenviromental Importance of Dinosaur Footprints, Soc. Sediment. Geol., 1, 37–47, https://doi.org/10.2307/3514457, 1986.
Lockley, M. G.: Therapod- and Prosauropod-Dominated Ichnofaunas from the Navajo-Nugget Sandstone (Lower Jurassic) at Dinosaur National Monument: Implications for Prosauropod Behavior and Ecology, New Mexico Museum of Natural History and Science, 316–320, 2011.
Lovejoy, E. M.: Geology of Cerro Rey Upflit, Chihuahua and New Mexico, New Mexico Institute of Mining & Technology, 92 pp., 1976.
Martinez, V. V.: Reconstructing Dinosaur Foot Tracks and Identifying New Dinosaur Footprints using Structure from Motion Photogrammetry, MS Thesis, U. of Texas at El Paso, 2016.
Pavlis, T. and Mason, K.: The new world of 3D geologic mapping, GSA Today, 27, 4–10, https://doi.org/10.1130/GSATG313A.1, 2017.
Remondino, F., Rizzi, A., Girardi, S., Petti, F. M., and Avanzini, M.: Ichnology-Recovery Digital 3D Models of Dinosaru Footprints, The Photogrammetric Records, 25, 266–282, 2010.
Rotzien, J. R., Sincavage, R., Pellowski, C., Gavillot, Y., Filkorn, H., Cooper, S., Shannon, J., Yildiz, U., Sawyer, F., and Uzunlar, N.: Field-Based Geoscience Education during the COVID-19 Pandemic: Planning, Execution, Outcomes, and Forecasts, GSA Today, 31, 4–10, https://doi.org/10.1130/GSATG483A.1, 2021.
Tavani, S., Granado, P., Corradetti, A., Girundo, M., Iannace, A., Arbues, P., Munoz, J. A., and Mazzoli, S.: Building a virtual outcrop, extracting geological information from it, and sharing the results in Google Earth via OpenPlot and Photoscan: An example from the Khaviz Anticline (Iran), Comput. Geosci., 63, 44–53, https://doi.org/10.1016/j.cageo.2013.10.013, 2014.
Thulborn, T.: Dinosaur tracks, xvii Edn., Chapman & Hall, London, 1990.
Walker, J., Tikoff, B., Newman, J., Clark, R., Ash, J., Good, J., Bunse, E. G., Moller, A., Kahn, M., Williams, R. T., Michels, Z., Andrew, J. E., and Rufledt, C.: StraboSpot data system for structural geology, Geosphere, 15, 533–547, https://doi.org/10.1130/GES02039.1, 2019.
Wenzel, K., Rothermel, M., Fitsch, D., and Haala, N.: Image Acquisition and Model Selection for Multi-View Stereo. International Archives of the Photogrammetry, Remote Sensing and Spatial Information Sciences, XL-5(W1), 251–258, https://doi.org/10.5194/isprsarchives-xl-5-w1-251-2013, 2013.
Westoby, M. J., Brasington, J., Glasser, N. F., Hambrey, M. J., and Reynolds, J. M.: Structure-from-Motion photogrammetry: A low-cost, effective tool for geosicence applicaitons, Geomorphology, 179, 300–314, https://doi.org/10.1016/j.geomorph.2012.08.021, 2012.
Whitmeyer, S., Pyle, E., Pavlis, T., Swanger, W., and Roberts, L.: Modern approaches to field data collection and mapping: Digital methods, crowdsourcing and the future of statistical analyses, J. Struct. Geol., 125, 29–40, https://doi.org/10.1016/j.jsg.2018.06.023, 2019.
Whitmeyer, S., Atchison, C., and Collins, T.: Using mobile technologies to enhance accessibility and inclusion in field-based learning, GSA Today, 30, 4–10, https://doi.org/10.1130/GSATG462A.1, 2020.
realscience.