the Creative Commons Attribution 4.0 License.
the Creative Commons Attribution 4.0 License.
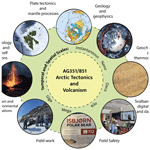
Arctic Tectonics and Volcanism: a multi-scale, multi-disciplinary educational approach
Grace Shephard
Fenna Ammerlaan
Owen Anfinson
Pascal Audet
Bernard Coakley
Victoria Ershova
Jan Inge Faleide
Sten-Andreas Grundvåg
Rafael Kenji Horota
Karthik Iyer
Julian Janocha
Morgan Jones
Alexander Minakov
Margaret Odlum
Anna Sartell
Andrew Schaeffer
Daniel Stockli
Marie Annette Vander Kloet
Carmen Gaina
Geologically, the Arctic is one of the least-explored regions of Earth. Obtaining data in the high Arctic is logistically, economically, and environmentally expensive, but the township of Longyearbyen (population of 2617 as of 2024) at 78° N represents a relatively easily accessible gateway to Arctic geology and is home to The University Centre in Svalbard (UNIS). These unique factors provide a foundation from which to teach and explore Arctic geology via the classroom, the laboratory, and the field. UNIS was founded in 1993 as the Norwegian “field university”, offering field-based courses in Arctic geology, geophysics, biology, and technology to students from Norway and abroad.
In this contribution, we present one of the educational components of the international collaboration project NOR-R-AM (a Norwegian-Russian-North American collaboration in Arctic research and collaboration, titled Changes at the Top of the World through Volcanism and Plate Tectonics) which ran from 2017 to 2024. One of the key deliverables of NOR-R-AM was a new graduate (Master's and PhD-level) course called Arctic Tectonics and Volcanism that we have established and taught annually at UNIS since 2018 and detail herein. The course's main objective is to teach the complex geological evolution of the Arctic from the Devonian period (∼ 420 million years ago, Ma) to the present day through integrating multi-scale datasets and a broad range of geoscientific disciplines. We outline the course itself before presenting student perspectives based on both an anonymous questionnaire (n=27) and in-depth perceptions of four selected students. The course, with an annual intake of up to 20 MSc and PhD students, is held over a 6-week period, typically in spring or autumn. The course comprises modules on field and polar safety, Svalbard/Barents Sea geology, wider Arctic geology, plate tectonics, mantle dynamics, geo- and thermochronology, and geochemistry of igneous systems. A field component, which in some years included an overnight expedition, provides an opportunity to appreciate Arctic geology and gather field observations and data. Digital outcrop models, photospheres, and tectonic plate reconstructions provide complementary state-of-the-art data visualization tools in the classroom and facilitate efficient fieldwork through pre-fieldwork preparation and post-fieldwork quantitative analyses. The course assessment is centred around an individual research project that is presented orally and in a short and impactful Geology journal-style article. Considering the complex subject and the diversity of students' backgrounds and level of geological knowledge before the course, the student experiences during this course demonstrate that the multi-disciplinary, multi-lecturer field-and-classroom teaching is efficient and increases their motivation to explore Arctic science.
- Article
(16540 KB) - Full-text XML
- BibTeX
- EndNote
The Arctic is considered one of the last geoscientific frontiers in the world (Fig. 1). This is partly a consequence of the region being relatively poorly mapped (a result of accessibility difficulties) and compounded by the debate around its geological evolution. The Arctic offers geological diversity encompassing onshore and offshore environments and includes active subduction zones in Alaska, deep sedimentary basins on the Siberian and Barents Sea shelves, widespread ancient volcanism and magmatism, the world's slowest-spreading mid-ocean ridge (Gakkel Ridge in the Eurasia Basin), and world-class examples of extensional and compressional basins exposed on Svalbard (Fig. 2). The centre of the Arctic is a deep oceanic basin, which is surrounded by shallower continental shelves of variable widths (Fig. 1). The onshore domains are divided socio-politically into five coastal nations and an additional three when considering countries north of the Arctic Circle (66°34′ N). The Arctic is home to approximately 1 million indigenous people that represent ca. 9 % of its total population (Nordregio, 2019). The physiographic configuration underpins much of the climatic, oceanographic, biological, and sociopolitical development of the region in both the present day and recent past (hundreds to hundreds of thousands of years ago) and deep time (millions of years ago).
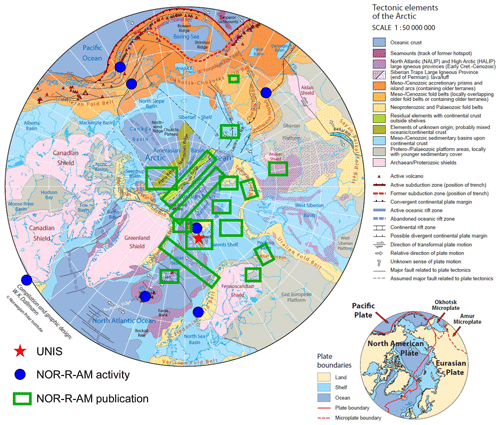
Figure 1Tectonic elements map of Arctic. The red star marks the location of the University Centre in Svalbard (UNIS). The base map is from Dallmann (2015). The blue circles indicate the location of NOR-R-AM activities as listed in Table 4. The green boxes indicate the approximate location of NOR-R-AM-affiliated publications (Senger and Galland, 2022; Prokopiev et al., 2018, 2019; Ershova et al., 2022; Rogov et al., 2023a, b, 2017; Vasileva et al., 2022; Abdelmalak et al., 2023; Abdelmalak et al., 2024; Nikishin et al., 2018; Anfinson et al., 2022; Brustnitsyna et al., 2022; Ershova et al., 2018; Khudoley et al., 2019; Struijk et al., 2018; Døssing et al., 2020; Straume et al., 2020; Straume et al., 2022; Gaina, 2022; Blischke et al., 2022; Døssing et al., 2017; Kurapov et al., 2021; Lebedeva-Ivanova et al., 2019).
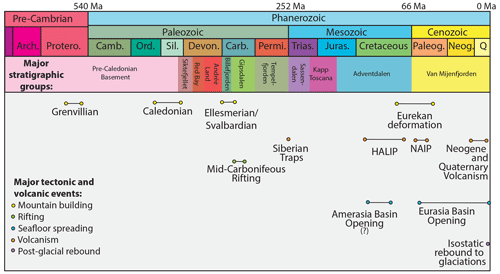
Figure 2Summary chart of the major stratigraphic groups in Svalbard (note that regional hiatuses exist in the Upper Cretaceous and the Neogene) and the major tectonic and volcanic events affecting the area. HALIP: High Arctic Large Igneous Province. NAIP: North Atlantic Igneous Province.
The effects of climate change, notably manifested as a global temperature increase, is up to 4 times greater in the Arctic region than other regions of the world due to polar amplification (Serreze and Barry, 2011). Consequently, the Arctic demands immediate attention and a concentration of efforts in order to prepare for the coming years and decades and beyond. It is inevitable that there will be increased human activity in the Arctic, for example, through a combination of natural resource extraction, tourism, shipping, and fisheries. Global attention from the broader public is increasingly turning to the Arctic and is reflected in the media, governmental policies, and educational resources (Ford et al., 2021; Heininen et al., 2020). An opportunity for geoscientists to contribute to this is through understanding and communicating the various driving forces that have created the features of the region, including how the region has undergone changes in the past and how it will likely respond to various forcing factors in the future.
Characterizing the present-day structure of the Arctic Ocean basin and its surrounding submerged continental shelves relies on reconciling multi-physical datasets collected from multiple platforms, including scientific vessels, aeroplanes, and satellites and using various technologies to take liquid, gas, and rock samples from the seafloor and subsurface. For example, Kristoffersen (2011) synthesized the geophysical exploration of the Arctic Ocean, pointing to the challenges of thick sea ice during data acquisition and the necessity for Arctic-specific platforms like sub-ice submarines and drifting ice stations like the German-led MOSAiC icebreaker drift (Krumpen et al., 2021) or the hovercraft-based Fram-2014/15 (Kristoffersen et al., 2023) expeditions. Over the past decade, exponentially more Arctic data have been acquired. This was partly facilitated by the diminishing sea ice and partly by geopolitical considerations related to the United Nations Convention on the Law of the Seas (UNCLOS; Proelss, 2009). Through UNCLOS, the states geographically bordering the Arctic Ocean could apply to extend their continental shelf towards the central Arctic Ocean. Brekke and Banet (2020) outline the procedure from the Norwegian perspective, the maritime territorial margins of which have been recently settled.
Understanding the geological evolution of the Arctic basin itself relies largely on deciphering the geology of the surrounding landmasses such as Svalbard. Historically, a lot of Arctic research was conducted along N–S gradients – by Norwegians in Svalbard, Danes in Greenland, Swedes and Finns in northern Fennoscandia, Russians in Siberia, US Americans in Alaska, and Canadians in Arctic Canada. The same could be said for the tertiary educational systems, where students often study in their country or continent of origin. In contrast, Arctic indigenous students often move from their homes to study outside the Arctic as most higher education institutes are located south of the Arctic Circle. Svalbard, however, has no indigenous population, and all UNIS students have to migrate north to undertake studies there. However, such a latitudinal and unilateral framework limits a true system-wide perspective and the basic and applied scientific discoveries that come with it. A circum-polar approach also involving multi-national partners from non-Arctic nations with significant Arctic research programmes (e.g. Germany, South Korea, and China) is required. Furthermore, to understand the deep time evolution of this part of the world and its place in the global system, a wide range of geoscience disciplines needs to be integrated. Ideally, research and educational projects should reflect this by spanning both spatial and temporal scales.
A limiting factor of conducting research in the Arctic are the financial costs. This is particularly true for scientific field campaigns that are conducted via either land, sea, air, or space. Universities and academic institutions (funded either internally or via external funding agencies) thus take a deliberate and measured approach to acquire funding to support such activities. Another consideration is the carbon footprint related to travel to and from the Arctic, with many researchers becoming increasingly aware of the balance between climate change and its impacts on the environment and undertaking the research and knowledge transfer about it (e.g. Topp-Jørgensen et al., 2022). In addition to research, the role of education (here considering tertiary-level courses) and student mobility to and from (and within) the Arctic is crucial. The intersection of scientific research and education in the Arctic is thus an emerging opportunity.
Several pedagogical-meets-research approaches or activities have been recently implemented regarding Arctic and polar science. For the geosciences, these include a high school classroom implementation of the Arctic Climate Connections curriculum (Gold et al., 2015) a special volume focussing on polar education (Gold et al., 2021), and insights into developing a field course in Arctic glaciers and landscapes (Malm, 2021).
The University Centre in Svalbard (UNIS; Fig. 1) is a unique educational institution. It is a shareholding company fully owned by the Norwegian Ministry of Education and Research and does not charge tuition fees. It was established in 1993 to provide university-level education in Arctic studies, to carry out high-quality research, and to contribute to the development of Svalbard as an international research platform. UNIS offers undergraduate-, graduate-, and postgraduate-level courses, all delivered in the English language. One example of the integration of research and education has been presented by Senger et al. (2021b), who focussed on an annual BSc-level course taught at UNIS, titled Integrated Geological Methods: from outcrop to geomodel. The course had been partially externally funded through the University of the Arctic (UArctic) and paved the way for the development of the Svalbox digital outcrop model (DOM) database (Betlem et al., 2023; Senger et al., 2021a). Svalbox facilitates further activity including MSc and PhD projects that systematically contribute to the growing database of DOMs.
UNIS courses require a field component, thus maximizing the benefit of being located in Svalbard, the natural laboratory of the high Arctic. With this in mind, no UNIS course could be held at universities on the Norwegian mainland, and UNIS is thus considered Norway's field university. This is reflected amongst others in ongoing work as part of two Norwegian Centres for Excellence in Education, bioCEED and iEarth, where UNIS is actively involved, particularly with field teaching and learning. Some of this work reflects the increased use of digital tools such as digital field notebooks (Senger and Nordmo, 2021) or virtual field guides (Eidesen and Hjelle, 2023) to bring the classroom to the field sites and incorporate student accessibility and inclusivity. Malm (2021) provides a more pedagogical approach focussing on a graduate course on Arctic glaciers and landscapes. Nonetheless, a truly circum-Arctic approach that integrates the spatial and temporal range required to decipher the deep-time evolution of the Arctic region is currently missing from the curriculum of Norwegian university courses. Secondly, there are few publicly available thematic datasets that have been pre-compiled and allow for the multi-disciplinary teaching of Arctic geology.
In this contribution we present an international collaboration project, NOR-R-AM (a Norwegian-Russian-North American collaboration in Arctic research and collaboration, titled Changes at the Top of the World through Volcanism and Plate Tectonics). In particular, we focus on describing a direct outcome of this project, which was a 10-ECTS (European Credit Transfer and Accumulation System) MSc- and PhD-level course jointly developed by project members. The 6-week course was held at UNIS and was titled Arctic Tectonics and Volcanism. The annual course has run consecutively from 2018 onwards. Throughout the course, integration across temporal and spatial scales across disciplines and across various software tools is stressed as an important learning objective. One of the key motivations of this contribution is to provide adequate information, expert insights, and data packages so that the course can also be taught (albeit without the field component) elsewhere. As such, we also provide two quality-controlled datasets along with teaching material so that these datasets, and/or their formulations, can be implemented elsewhere, whether it be in Arctic, polar, geoscience, or other courses.
NOR-R-AM is the acronym for the project NOR-R-AM, a Norwegian–Russian–North American collaboration in Arctic research and collaboration. There were two successive generations of the project, NOR-R-AM and NOR-R-AM2, which were funded for the periods of 2017–2019 and 2020–2024, respectively. The projects were awarded funding from the Norwegian Research Council under the INTPART call (International Partnerships for Excellent Education, Research and Innovation) and from SIU, the Norwegian Centre for International Cooperation in Education (now DIKU). At the time of the calls (2016 and 2019, respectively), partnerships for this call were possible with circum-Arctic nations USA, Canada, and Russia, and the NOR-R-AM project formed as a collaboration with world leading groups in Arctic geosciences, including
-
University of Oslo, Norway (project lead);
-
University Centre in Svalbard (UNIS), Norway;
-
University of Alaska Fairbanks (UAF), USA;
-
University of Texas (UTIG), USA;
-
Sonoma State University, USA;
-
Natural Resources Canada;
-
University of Ottawa, Canada;
-
Saint Petersburg State University, Russia (active partner until February 2022);
The aim of the NOR-R-AM project was to set a scientific basis for deciphering the timing, driving forces, and consequences of volcanism in the Arctic region. While educating the next generation of Arctic experts, this international collaboration also prepared a scientific platform for future large, collaborative research initiatives in the Arctic. Six work packages (WPs) were established, namely onshore geology (WP1), offshore geology (WP2), Arctic seismicity and deep interior (WP3), arctic volcanism and palaeo-environment (WP4), circum-Arctic geodynamics (WP5), and education (WP6), each led by a partner institution. In this contribution, we focus on the WP6, education, with emphasis on the course we jointly developed and have taught at UNIS since 2018.
The Arctic Tectonics and Volcanism course (AG-x51) was a key deliverable of the NOR-R-AM project and is offered at UNIS simultaneously as both an MSc-level (course code AG-351) and a PhD-level (AG-851) course. This course addresses the diverse geological history of the Arctic region, including both onshore and offshore regions from Paleozoic to recent times (over 500 million years of history). This can be described as a 4-dimensional perspective because it looks at the surface, deep interior, present day, and deep past. The course focusses on the interplay of plate tectonics (including mountain building processes of rifting, seafloor spreading, subduction, and orogenesis) and (arc, rifting and plume-related) volcanism across several scales (Fig. 3). It explores some of the outstanding questions within the Arctic research community with regional case studies, scientific datasets, and state-of-the-art software programs and methodologies. Based at the gateway to the Arctic, Svalbard (Fig. 1), the course is complemented by several field excursions, which examine the well-exposed outcrops and specifically the igneous rocks emplaced over large parts of the Svalbard Archipelago.
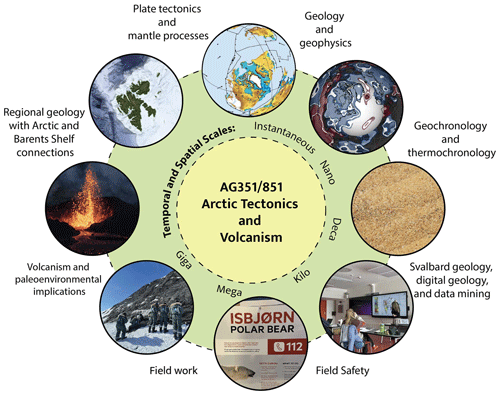
Figure 3Synthesis of the Arctic Tectonics and Volcanism course with the main modules covered. The course addresses heterogeneity laterally (Svalbard–Barents Shelf–Arctic–depth–global), in depth (shallow to deep processes), and across spatial and temporal scales (from nanoscales through to global maps or gigascales). The maps are produced from the compiled thematic datasets provided by Senger and Shephard (2023).
Entry requirements for this Master's and PhD course are the pre-existing enrolment in a relevant Master's and PhD programme, respectively, anywhere in the world. A general background in structural geology, sedimentology, volcanology, geodynamics, or geophysics was encouraged, and previous geological field experience was advantageous, though not necessary. Many of the students who attended had little or no experience in Arctic geology nor polar fieldwork.
As with all higher education in Norway, the course has no tuition fee. Students only need to pay a semester fee (ca. EUR 50) in order to sit all exams during a semester and contribute ca. EUR 20 per day towards food for overnight excursions. The NOR-R-AM project fully funded the tuition fees, travel, and accommodation for students from affiliated institutions and the overnight food contribution to all students. The harsh Arctic weather conditions, strong seasonality, and several fieldwork-related hazards (mitigated by targeted training) all contribute to a unique study experience. The diverse society in Longyearbyen is inclusive, caring, and safe irrespective of gender, sexual orientation, or nationality. Schengen transit visas are required for travel to and from Svalbard through Norway, but due to the Svalbard Treaty, there are no visa requirements to study, live, or work in Svalbard itself.
The course is intentionally scientifically and geographically broad but is limited by the 6-week period applicable to 10-ECTS intensive courses. Financially, the biggest budget posts are field activities and travel/salary costs for the significantly higher number of guest lecturers compared to other UNIS courses. From a pedagogical perspective, students attending the AGx51 course (and other UNIS courses too) typically have a varied background reflecting diverse home universities and study programmes. On the one hand, this requires careful consideration for the teaching staff regarding balancing the academic content. On the other hand, we consider this heterogeneous background as beneficial in the context of “experts in a team” as students are placed in groups reflecting their complementary expertise and encouraged to also teach each other.
Development and modification of the Arctic Tectonics and Volcanism course has continually occurred since the 2-week long pilot course was held in 2018. Annual course evaluations from the students were considered when optimizing the course. The primary elements of the course development can be broadly summarized as follows:
-
The overall course curriculum is in line with UNIS and Norwegian University accreditation.
-
The individual lectures are conducted by a large team of scientists from different career stages in academia and industry, which included theoretical and practical components.
-
The course included fieldwork, with single-day site visits and multi-day field trips involving multiple transport options (via sea and land, including using snowmobiles). The locations visited were dependent on the time of year that the course was run (spring, summer, and autumn); the availability of transport and logistics; cost; and, finally, the weather and safety conditions on the day.
-
The goal remains to promote outreach and science communication with both the community in Svalbard and more widely.
The learning outcomes were designed to that, upon completing the course, the students will gain specific knowledge, skills, and general competencies.
-
Knowledge. These include
-
understanding the physical, chemical, and structural characteristics of volcanic provinces onshore and offshore;
-
being able to understand tectonic plate principles;
-
being aware of the links between surface and deep mantle, methods and models using various data including seismology and satellite data;
-
understanding the causal connections between tectonic evolution and episodic bursts of volcanism as well as the impacts volcanism can have on the global climate.
-
-
Skills. These include
-
knowing how to identify first-order tectonic provinces from geophysical and geological data;
-
being able to make a first-order interpretation of geophysical, geochemical, and geological data connected to magmatic provinces;
-
being able to make tectonic plate reconstructions using modern software;
-
being able to interpret mantle tomography models and integrate them in large-scale tectonic interpretation;
-
being able to identify and characterize igneous rocks in the field;
-
being able to discuss how igneous plumbing systems may affect subsurface fluid migration.
-
-
General competencies. These include
-
gaining firsthand experience of actively working both individually and in small groups,
-
learning how to effectively and safely undertake fieldwork in Arctic conditions,
-
improving the presentation skills by presenting their work to their peers and creatively tackling the set problems,
-
communicating their research findings through an article-style report.
-
The course components were spread across eight topic-based modules (Arctic geology and geophysics; Arctic plate tectonics and mantle processes; regional geology and Arctic and Barents Shelf connections; Svalbard geology, digital geology, and data mining; geochronology and thermochronology; volcanism and palaeo-environmental implications; field safety; and fieldwork), which are described below in terms of major outstanding regional questions, key datasets, and/or software.
4.1 Arctic geology and geophysics
Teaching about circum-Arctic geology and geophysics within a single lecture (typically 1.5 h long with a 15 min break) is a challenging task and was aided by the student cohorts having a good geoscience background from their undergraduate studies. A particular challenge was to capture the most relevant and up-to-date information about this vast topic and to prepare the students for understanding other aspects of Arctic's structure and evolution during the rest of the course. In the first teaching year (2018), when the course was very short (10 d), the tour de force lecture presented what is known about the region's surface and subsurface by reviewing the latest knowledge and the key datasets used. A central role in this presentation was played by showing how an international circum-Arctic mapping project gathered most of the updated information held by the Arctic nations (Norway, Russia, USA, Canada, Finland, Sweden, and Denmark) for building geological, tectonic, and geophysical maps of the Arctic (Fig. 4; Gaina et al., 2011; Petrov et al., 2021). The students learned that collecting geoscientific data in the Arctic is difficult and expensive and that wide collaboration with other countries and scientists is essential for advancing the knowledge of this remote region.
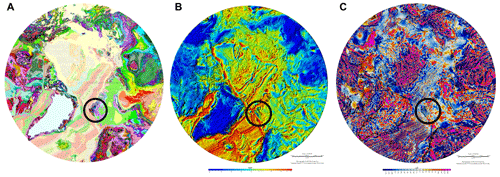
Figure 4Maps showing the (a) geology (Harrison et al., 2008) and geophysics (Gaina et al., 2011) as a (b) gravity anomaly and (c) magnetic anomaly of the circum-Arctic region. The black circle shows Svalbard and the surrounding area.
The lecture also emphasized the important role of remote sensing data, and especially satellite data (including gravity and magnetic anomalies), for deciphering the Arctic crustal and lithospheric structure. In addition, it presented the role of the upper and lower mantle and their heterogeneities in the Arctic's tectonic and magmatic evolution and how mantle structures could be identified using tomographic models obtained from seismological data. With a field excursion organized later in the course, this foundational lecture briefly mentioned how new geophysical data contribute to refined tectonic models of Svalbard and the surrounding Barents Sea.
The pedagogical approach for the course was modified for subsequent years (2019, 2022, 2023, and 2024), when the course was offered for a longer period (6 weeks) and the students had more time to consult the recommended bibliography. The next iterations of the Arctic geology and geophysics lecture(s) explored the understanding of this region by presenting the main tectonic features according to their ages, from oldest (cratons) to the youngest (oceanic basins). Relevant methods for assessing their structure and ages, with an emphasis on geophysical methods, were presented, and, when possible, examples including Svalbard and surrounding regions were given. In a computer practical, the students were also introduced to the free application GeoMapApp (https://www.geomapapp.org, last access: 6 November 2024; Ryan et al., 2009) used to display and analyse geoscientific datasets. We made sure that the geological connections between land and sea and among various Arctic sub-regions were presented in the regional (and even) global context and that the latest published studies featured in the presentation, with many studies also included in the reading list. The lecture was usually wrapped up by informing the future Arctic scientists about the work in progress, the need for future studies, and the opportunities for student involvement in projects such as NOR-R-AM. Because there were several guest lecturers in attendance throughout the course, the students had the opportunity to discuss and ask more detailed questions about active research and outstanding questions.
4.2 Arctic plate tectonics and mantle processes
Tectonics is a core theme of this course, and the various links between tectonic processes such as ocean basin opening, mountain building, subduction, sedimentary basin formation, and volcanism and magmatism (including rift- and mantle-plume-related events). The links to climatic, oceanographic, and biogeographic changes are mentioned throughout the lectures. Many of the students had already been introduced to the concept of plate tectonics; nonetheless, this module includes a set of introductory and more advanced lectures. Following on from a refresher about plate tectonics at the global scale, Arctic-specific tectonics were then delivered by dividing history into three time periods (which could be presented running either forwards or backwards in time), the Cenozoic, Mesozoic, and Paleozoic. These three time periods cover the major Arctic tectonic events, including but not being limited to North Atlantic and Eurasia Basin opening and Eurekan deformation (Cenozoic), Amerasia Basin opening and Pacific subduction (Mesozoic), and Ellesmerian deformation (Paleozoic). These events were discussed in terms of their influence on the regional to local tectonic expressions and influence on sedimentation.
In addition to theory-based lectures, several hands-on computer tutorials about viewing, analysing, and modifying plate reconstructions were undertaken over 2–3 sessions. These tutorials are based on the widely used and open-source plate tectonics software GPlates (Müller et al., 2018; Boyden et al., 2011), which was installed either directly on the students' personal laptops or on the desktop machines in the UNIS computer lab. In addition to the default files shipped with GPlates, the students were provided with an Arctic dataset bundle (Senger and Shephard, 2023), which included vector and raster data specific to the Arctic and had been published in peer-reviewed articles by the wider Arctic community, which could be easily loaded into GPlates. The data include regional gravity and magnetics and derivatives (Saltus et al., 2011; Gaina et al., 2011), crustal thickness maps (Lebedeva-Ivanova et al., 2019), seismic tomography models (Schaeffer and Lebedev, 2013; Ritsema et al., 2011), and bathymetry (Jakobsson et al., 2012). The students were taught about the mathematical method to rotate rigid entities on a sphere; the Euler and finite rotations; how to view and display plate reconstructions and related data, including spreading rates and motion paths; and how to change frames of reference (absolute and relative), import and export data and images, and make animations of tectonic motions through time.
Because plate tectonics is the surface manifestation of a convecting mantle, it is also relevant to explore the structure and evolution of the deeper Earth interior. It is also particularly relevant for the discussion of large-scale volcanism because their emplacements are linked to the arrival of deep-seated mantle plumes that rise throughout the mantle and erupt at the surface. Such major volcanic and large igneous provinces of the Arctic include Iceland, the Paleogene North Atlantic Igneous Province (NAIP), the Cretaceous High Arctic LIP (HALIP), and the Permian Siberian Traps LIP. In a set of at least two lectures, the mantle is discussed from the perspective of what the major features that contribute to its heterogeneities are (including subducted slabs and plumes and core–mantle boundary features), what the main geophysical methods and datasets allowing for the identification of these mantle structures are (including gravity and the geoid, seismic tomography, and numerical modelling and geochemistry), and what the potential role of mantle dynamics in the enigmatic origins of the long-lived and pulsed HALIP is. As part of this lecture, the community-based visualization website SubMachine (Hosseini et al., 2018) was shown to the students, who learned how to plot and analyse different seismic tomography models both globally and specifically for the Arctic region.
4.3 Regional geology with Arctic and Barents Shelf connections
Svalbard plays a critical role in our understanding of the tectonic and palaeogeographic evolution of the Arctic. In this module, we explore the regional geologic setting of Svalbard and delve into the tectonic events that have shaped Svalbard by placing those tectonic events into the larger tectonic evolution of the circum-Arctic. This begins by introducing the students to the major continental blocks that surround the present-day Arctic (i.e. Baltica, Laurentia, and Siberia; Fig. 6a) and to some of the currently exposed continental fragments within the Arctic (e.g. Svalbard, Franz Josef Land, New Siberian Islands, and Wrangel Island) that lend insight into the overall tectonic and palaeogeographic framework of the Arctic (e.g. Blakey, 2021). We then focus on the Neoproterozoic and younger mountain building events (e.g. Timanian, Caledonian, Ellesmerian/Svalbardian, Uralian, and Eurekan mountain belts) that have either influenced the tectonic structure of Svalbard or been a major sedimentary source for sedimentary successions exposed in Svalbard. This section of the course culminates with a focus on detrital zircon geochronologic datasets, time markers that allow for establishing the age of major tectonic events, that have been collected from Paleozoic sedimentary strata across the Arctic. The students also learned how data collected (as part of this course) from Svalbard have aided our understanding of Svalbard's regional tectonic evolution (Anfinson et al., 2022; Fig. 5).
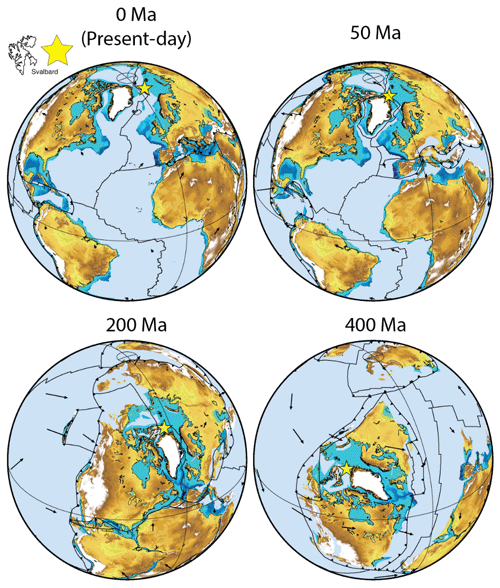
Figure 5Tectonic plate reconstruction of Svalbard (located at the yellow star) and the Arctic in the global tectonic setting at present day (0 million years, Ma), 50 Ma (opening of the Eurasia Basin and North Atlantic), 200 Ma (pre-opening Amerasia Basin), and 400 Ma (Arctic located in equatorial latitudes). These are based on the global plate reconstruction of Müller et al. (2018) constructed from regional studies and made using the GPlates software. Present-day topography and bathymetry (cut to continental domains, light blue in oceans) are for reference only.
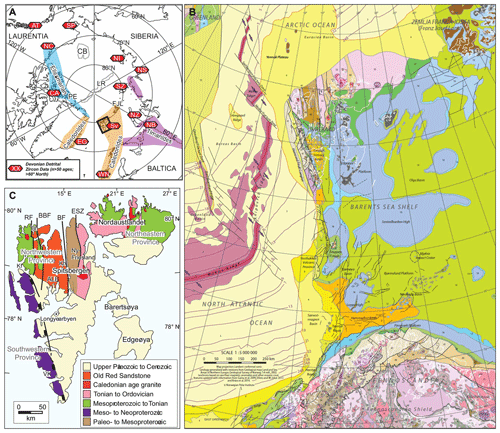
Figure 6(a) Schematic tectonic map of the modern Arctic depicting the general location of the Timanian, Caledonian, and Ellesmerian mountain belts. Symbols indicate the location of Devonian detrital zircon data (see Anfinson et al. (2022); map adapted from Colpron and Nelson, 2009). The detrital zircon locations are east Greenland (EG), Canadian Arctic Islands (CA), NC, northwest Canada (NC), Alexander terrane (AT), Seward Peninsula (SP), New Siberian Islands (NI), northeast Siberia (NS), Severnaya Zemlya (SZ), Novaya Zemlya (NZ), northern Baltica (NB), Svalbard (Sv), and western Norway (WN). The geographic references are Pearya terrane (PE), Lomonosov Ridge (LR), Chukchi Borderland (CB), and Franz Josef Land (FJL). (b) Setting of Svalbard on the interface between the North Atlantic and Arctic oceans and the rest of the Barents Shelf; the figure is from Dallmann (2015). (c) Geologic terrane map of Svalbard showing the location of the northeastern, northwestern, and southwestern provinces. The tectonic elements are Billefjorden Fault Zone (BF), Breibogen Fault (BBF), Raudfjorden Fault (RF), Eolussletta Shear Zone (ESZ), the Vimsodden–Kosibapasset shear zone (VK), and the Andre Land Basin (ALB). The geographic references are Kronprinshoegda (Kh), Kongsfjorden (Kf), and Biscayarhalvoya (Bh). The map is adapted from Beranek et al. (2020) and based on the geologic map of Gee (2015).
To the south and east, Svalbard is directly connected to the submerged parts of the Barents Shelf (Fig. 6b). Geoscientists involved in ongoing petroleum exploration and production as well as active CO2 storage in the southwestern Barents Shelf use Svalbard as an excellent analogue to the reservoirs and cap rocks further south (Olaussen et al., 2024; Henriksen et al., 2011). The region is naturally rich in exploration wells and seismic data, with a much denser coverage than on Svalbard. Not only are these data used to constrain the reservoir extent and architecture, but they also understand the larger-scale trends. Notable examples include detailed characterization of major sedimentary wedges in the Triassic and Cretaceous. The Triassic system, representing the largest delta plain in Earth's history (Klausen et al., 2019), is a westerly prograding system seen as clinoforms in seismic data across the Barents Shelf (Glørstad-Clark et al., 2011; Gilmullina et al., 2021) and as sand-prone sediments on Svalbard (Anell et al., 2014; Lundschien et al., 2014). The Cretaceous system is linked to uplift to the north associated with the HALIP emplacement, regional tilting, and fluvial-dominated system traversing Svalbard from the north depositing sediments to the south (Midtkandal et al., 2019; Grundvåg et al., 2017).
4.4 Svalbard geology, digital geology, and data mining
The Svalbard Archipelago, with its polar climate, offers vegetation-free and well-exposed outcrops testifying about a diverse tectono-stratigraphic evolution of the region. Geologically, Svalbard is presently the emergent part of the Barents Shelf but has been linked to Arctic Canada and northern Greenland prior to the Eurekan Orogen. The nearly continuous stratigraphic record from the Devonian to the Paleogene (Olaussen et al., 2024) provides evidence of Svalbard's overall northward motion through time overprinted by changing tectono-stratigraphic configurations. These include mid-Carboniferous rifting, Permian platform carbonates, Mesozoic siliciclastic deposits intruded by an igneous complex, and a Cenozoic fold-and-thrust-belt with an associated foreland basin. Late Cenozoic sediments are not present on Svalbard but occur in depocentres along the northern and western shelf margins off Svalbard.
However, Svalbard's high latitudinal position means that the rocks are snow-free and accessible only during a short summer season, typically from June to mid-September. During these times, boat-based transport and hiking is possible. Conversely, snow cover provides relatively easy access to large-scale inland outcrops via a snowmobile (that are too difficult to reach by foot) during the winter season with adequate light, from March to early May. The high seasonal dependence, combined with sudden weather events, has motivated us at UNIS to systematically acquire and openly share digital outcrop models (DOMs) and photospheres through the Svalbox database (Betlem et al., 2023; Senger et al., 2021a). These DOMs are georeferenced high-resolution 3-dimensional representations of the outcrops and facilitate quantitative sedimentological and structural work. Through Svalbox, the DOMs are also put in a regional context through spatial integration of maps (geological, topographical, palaeogeographic, geophysical, etc.), surface data (digital terrain models, satellite imagery, etc.), and subsurface data (boreholes, geophysical profiles, published cross sections, etc.) as illustrated for the Festningen geotope by Senger et al. (2022). Photospheres are systematically acquired as part of regular Svalbox campaigns and thematically grouped in virtual field trips using the VR Svalbard (https://vrsvalbard.com/, last access: 7 December 2024) platform (Horota et al., 2024). These drone-based 360° photographs provide a bird's eye perspective of the visited sites and are complementary to the more quantitative DOMs. Photospheres are also integrated into thematic datasets, for instance, related to the West Spitsbergen fold-and-thrust belt (Horota et al., 2023) visited during the October 2022 field campaign, to facilitate data access and the development of student projects. Students actively use these digital resources in the course to both prepare for fieldwork and conduct quantitative analyses as part of their individual research projects (Fig. 7).
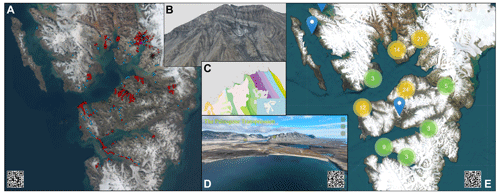
Figure 7Synthesis of key digital tools made available to the students. (a) Svalbox map (https://www.svalbox.no/map, last access: 6 November 2024) interface with digital outcrop models (blue dots) and photospheres (red dots). (b) An example of a digital outcrop model of compressional tectonics at Lagmannstoppen (Lord et al., 2021). The model was used as a basis for a research project in 2023. (c) Zoom-in to the Svalbox map interface across the famous geotype profile at Festningen. The geological layer is used as a base map. (d) Thematic virtual field trip of Paleogene transpression that has amongst others tilted the layers at Festningen. The field trip is related to a thematic dataset used in the course (Horota et al., 2023). (e) An overview of photosphere coverage in the VR Svalbard platform (https://www.vrsvalbard.com/map, last access: 6 November 2024; Horota et al., 2024) including photospheres specifically targeting the AG-x51 course.
4.5 Geochronology and thermochronology
Providing constraints on the absolute timing and duration of deformation as well as magmatic, metamorphic, or stratigraphic processes is of critical importance for deciphering the tectonic plate evolution of the Arctic. Radiometric dates serve as both input parameters and/or testable benchmarks for tectonic and thermal processes on all scales, from tectonic plate reconstructions and timing of magmatism to basin burial and maturation. The geochronology and thermochronology component of the AG-x51 course consists of three different learning modules: (1) overview and theory of radiometric dating methods, (2) application to tectonic and magmatic processes in the Arctic, and (3) hands-on exercises in detrital zircon U–Pb provenance analysis. The overview and theory for the geochronology portion covered the basics of radioactive decay, including which long-lived isotopes undergo radioactive decay and are commonly used in earth science applications, half-lives, how we can use the measured daughter and parent isotope ratios to calculate an age, and what makes a good mineral or system to use (i.e. radioactive parent with a well-defined half-life, no non-radiometric daughter isotope at t=0, mineralogic stability, etc.). We then focussed on U–Pb geochronology, which is widely applied everywhere, including the Arctic, and how we measure, calculate, plot, and evaluate U–Pb ages.
We discussed how U–Pb chronology can be applied to a wide suite of Arctic questions with specific examples including dating HALIP around the Arctic (Evenchick et al., 2015; Corfu et al., 2013) and palaeogeographic reconstructions using detrital zircon U–Pb provenance (Anfinson et al., 2012). The thermochronology portion introduced thermally activated diffusion and closure temperature (Dodson, 1973) as they apply to noble gas thermochronology (i.e. Ar–Ar and (U–Th) He systems) and the basic principles of fission track thermochronology. We discussed the differences in geo- and thermochronology and then the power or combining different methods to understand thermal and tectonic histories and discussed an example of using thermochronology to understand Eurekan deformation on Svalbard (Schneider et al., 2019). The students were then introduced to geochron.org, a public database for geo- and thermochronology data, and did various searches for data so they learned about resources to acquire and use available geochronologic data in their own projects.
4.6 Volcanism and palaeo-environmental implications
The climatic impacts observed from historical volcanic eruptions are well documented in the geological record, which allows us to assess the possible effects of elevated magmatic activity through time. Global climate is both dynamic and complex, and there is a plethora of ways that volcanic activity can influence local, regional, and global environmental conditions. This section of the course begins with an introduction to volcanism and magmatic systems, covering how melts are produced and how factors such as depth and degree of partial melting affect the melt composition. We then focus on the primary constituents of the melt and how this affects the physical properties of the magma, such as viscosity and saturation of volatile phases. We then follow the magmatic plumbing system towards the surface and investigate how these factors drive the style and explosivity of eruptions with the aid of a practical class. We end this section of the course by applying this information to outcrops in Svalbard, including the ash layers in the Palaeocene Firkanten Formation around Longyearbyen and also in Permian–Triassic sediments at Festningen. These layers act as marker horizons, which are used to help constrain plate reconstructions in the Arctic (Jones et al., 2017).
Once an understanding of volcanic processes has been established, we introduce the concepts of regional and global climate. This includes concepts such as the greenhouse effect and how changes in atmospheric concentrations of greenhouse gases affect the climate through time. We then take this information based on current observations into the palaeo-climate realm, covering what methods of proxy data are used to estimate palaeo-environmental conditions and at what timescales each of these proxies can be used. Once these key ideas are established, we focus on volcanism and how elevated activity can perturb the climate system. This includes emissions of climate-sensitive gases such as sulfur and carbon species and how different sources (e.g. volcanic degassing vs. emissions from contact metamorphism around shallow intrusions) have differing climatic impacts. We also consider post-eruption processes, such as the silicate weathering of volcanic ash as a significant atmospheric carbon sink. This section of the course is concluded by investigating examples of large-scale volcanic activity and environmental disturbances in the geological record in Svalbard, including the NAIP coeval with the Palaeocene–Eocene thermal maximum, the coincidence of HALIP and Cretaceous ocean anoxic events (OAEs), and the Siberian Traps being emplaced at the same time as the end-Permian mass extinction.
The role of contact metamorphism in large igneous provinces (LIPs) is manifold and is directly relevant for the Svalbard Archipelago. One of the most important effects of LIPs is the thermal impact of magma on the host rocks. The associated thermal maturation and/or cracking of organic matter found in sedimentary host rocks not only impacts hydrocarbon resources, but also was responsible for releasing massive quantities of greenhouse gases in the Earth's past, resulting in multiple mass extinction events (Svensen et al., 2004; Wignall, 2001; Hesselbo et al., 2002). Numerical modelling of these processes is an important tool to understand the effects they have on the environment and to also better constrain the physical parameters that drive them.
The course introduces the general physical processes and the associated equations that occur during magmatic emplacement. Basic modelling concepts and their advantages, uses, and caveats are outlined. A practical course that walks the students through the modelling of sill complexes with global examples and data from various LIPs is carried out using SILLi1D (Iyer et al., 2018), with a specific focus on HALIP magmatism in Svalbard (Brekke et al., 2014; Senger et al., 2014a). SILLi1D is an open-source, 1-dimensional finite-element (FEM) modelling tool that is specifically tailored to study the thermal effects of sill intrusions on the surrounding host rock. Model input is provided using MS Excel worksheets, which makes it accessible to a large audience with no previous programming skills. Input data are provided in the form of a simplified present-day well log or outcropping sedimentary column and include relevant rock parameters such as thermal conductivity, total organic carbon (TOC) content, porosity, and latent heats. Multiple sills can be emplaced within the system with varying ages and temperatures. Besides sill processes, the model also includes sedimentation and erosion, if any, to account for realistic basin evolution. The model output includes the thermal evolution of the sedimentary column through time and the host-rock changes that take place following sill emplacement such as TOC changes, thermal maturity (vitrinite reflectance), and amount of organic and carbonate-derived CO2. Rock parameters such as thermal conductivity and porosity are uncertain but only play a secondary role in controlling the overall thermal effects in a sill complex. The relative timing of sill emplacement together with emplacement temperature, however, exerts first-order thermal control in the aureole around a sill complex. These parameters are also not well constrained. The SILLi models can be used to better constrain such parameters if calibration data, such as vitrinite reflectance, are available by minimizing the error in the modelled results to the data. The amount of erosion also affects the background thermal maturity and can also be better estimated, similarly to sill emplacement parameters, by comparing the modelled maturity to the data. A number of examples are worked through with the students, with a few examples set aside as supervised exercises. The students are also encouraged to use the tool to investigate sill-complex outcrops from HALIP.
4.7 Field safety
Undertaking safe field operations is of paramount importance, and compulsory safety courses are held for all students and lecturers participating in the field component of the course. The courses are coordinated by the experienced safety and logistics staff of UNIS and are held over 2 to 3 full working days within the first week of the course. While experience is not something that can be trained, the strategy of the safety training is to give all participants irrespective of academic position the required skills to be able to make informed decisions about safety when in the field. Overall, 1 d is spent on safe rifle handling and polar bear encounter prevention, culminating in a practical shooting exercise at the rifle range outside Longyearbyen. The second day involves season-specific training; either survival suit or small-boat operations (for fieldwork in summer/autumn); or snowmobile safety and driving, sled packing, travel on sea ice/glaciers, and avalanche rescue (for fieldwork in spring). The third day includes first-aid techniques and navigation and communication protocols. Prior to undertaking any fieldwork (which by UNIS rules is defined as anything outside the UNIS building), briefings are held by all trip attendees and UNIS safety staff representatives. Before and during the fieldwork, plans were regularly adapted to account for weather conditions and wildlife sightings. As an example, the Paleogene basin infill sequence was only investigated from a distance, aboard the M/S Polarsyssel, in October 2023 due to a polar bear sighting in the area (Fig. 8d).
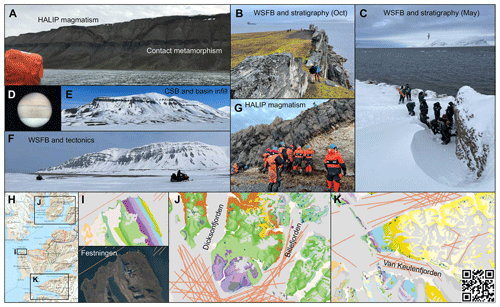
Figure 8Snapshots from the field component of the course. (a) Investigating a HALIP intrusion from a small boat and walking at Tshermakfjellet in July 2019. (b) Investigation of the Early Cretaceous Helvetiafjellet Formation at Festningen in October 2022. WSFB: West Spitsbergen fold-and-thrust belt. The Polarsyssel boat used as a base is in the background. (c) Fieldwork at the Festningen profile in late April 2023. (d) Polar bear seen through binoculars aboard the Polarsyssel, Van Keulefjorden, in October 2022. (e) Clinoforms in the infill sediments of the central Spitsbergen Basin, as seen from Reindalen, in late April 2023. (f) Scooter-based excursion to Isfjord Radio, late April 2023. Thrust tectonics related to the West Spitsbergen fold-and-thrust belt are seen on the Vardeborgsfjellet mountain in the background. (g) Investigation of the lower contact between a HALIP dolerite intrusion and Permian carbonate-dominated host rocks at Blomesletta, in October 2023. Note the survival suits used for safe access to this beach-side locality. (h) Overview map with key field sites investigated as part of the course, including Festningen (i), the Dicksonfjorden–Billefjorden area (j), and Van Keulenfjorden (k). Possible day excursions on snowmobiles from Longyearbyen are illustrated in (h). The base topographic map (h) is from the online open-access map from NPI, which is openly accessible at https://toposvalbard.npolar.no/ (last access: 6 November 2024). The base geological map (i, j, k) is from the Svalbox portal and openly accessible at https://www.svalbox.no/map (last access: 6 November 2024).
4.8 Fieldwork
Fieldwork is an integral part of all UNIS courses, and this course is also designed around a strong field component (Table 1; Fig. 8). The time of year and season(s) of when the course was run dictated the locations and operational requirements of the fieldwork and had to be adaptable to changing environmental conditions and any unforeseen logistical requirements at any time. Nonetheless, the fieldwork always tied the broader Barents Shelf and Arctic geology evolution to outcropping units that the students were describing and discussing as part of their field tasks. We detail selected field sites below, including the sites of Festningen, Diabasodden, the West Spitsbergen fold-and-thrust belt, the Central Spitsbergen Basin, and Billefjorden.
The Festningen profile, Svalbard's only geotope (i.e. an area formally protected because of geology), was visited in both late summer and early spring (one year experienced 0.5 m snowfall in the month of May). At Festningen, the students were able to visit and describe the main stratigraphic intervals and discuss correlations to the Barents Shelf and other Arctic basins. The entire section has been digitalized as a high-resolution DOM and integrated with geoscientific surface and subsurface data (Senger et al., 2022), which the students actively use in both preparing field stop preparations and post-fieldwork analyses.
Another key target for the field campaigns are the exposures of the Diabasodden Suite (Senger et al., 2014b; Dallmann et al., 1999), the local equivalents of the Early Cretaceous HALIP. The dolerites are exposed throughout Svalbard, and we often targeted the excellent exposures at Botneheia and Grønsteinfjellet. Here, both sills and dykes are well exposed and intersect a potential CO2 storage reservoir-cap rock system. This provides a theme for discussing how igneous plumbing systems affect subsurface fluid flow and also how the Svalbard dolerites correlate to the circum-Arctic HALIP.
Depending on the season and transport options, we also visit sites of relevance for tectono-stratigraphic evolution. These include the West Spitsbergen fold-and-thrust belt (the Svalbard part of the Eurekan mountain building event affecting large parts of the Arctic; Braathen et al., 1999; Piepjohn et al., 2016) and the associated foreland basin, the Central Spitsbergen Basin (Helland-Hansen and Grundvåg, 2020), as well as the mid-Carboniferous rift basin at Billefjorden (Smyrak-Sikora et al., 2019). Key sites that cannot be visited in person are addressed in lectures and using digital outcrop models and virtual field guides, including the WSFTB thematic data package provided by Horota et al. (2023).
All students must complete and pass the assessments to pass the course and receive the 10 ECTS. The assessment was broken into three components for the PhD-level students and two for the Master's students.
-
The first part was a pre-course assignment, which comprised an oral presentation of a scientific peer-reviewed paper which was presented to the class in the first weeks. The students could choose which paper to present from a set list provided before the start of the course. This presentation was worth 20 % of the final grade for the PhD students (0 % for the Master's students).
-
Another assessment was an oral presentation of a small research project in the final week of the class that had been developed throughout the course. This presentation was worth 20 % of the final grade for all students. The idea was that the students would be exposed to a range of Arctic geology topics, datasets, and software and would choose their proposed topic within the first 2 weeks, with ongoing guidance from someone from the lecturer team throughout the course. The students were encouraged not to simply choose a topic they may already be familiar with (or that formed their existing Master's or PhD-level thesis) but to use this opportunity to learn new skills and acquire knowledge.
-
Next, a Geology journal-style paper (four pages including figures, tables, and references) was handed in around 2 weeks after the course had concluded. This paper was based on the research project presented in point 2 and was worth 60 % of the final grade for PhD students and 80 % for Master's students.
-
The final grades were a letter grade from A (excellent) to F (fail) and delivered ca. 1 month after the end of the course. For the oral presentation of the projects, the students were given peer-evaluation forms to help provide constructive feedback to each other and encouraged to ask questions.
-
The research projects, with titles listed in Table 2, reflect the broadness of the course and also the students' own research interests. Numerous project ideas with associated datasets were made available, but students could also develop their own project ideas. Some of the projects, for instance, those looking into contact metamorphism studies using SILLi or tectonic plate reconstructions using GPlates, were directly tied to one of the course modules. Weekly update meetings with the lecturers were conducted with the students to ensure smooth progress. However, a lot of individual responsibility for time management was also strived towards to reflect the challenges of authentic life geologists will experience in future careers, be it in academia or the private sector.
The international approach that is needed for Arctic research was reflected in the participants of the course. Over the course of the years, students from educational institutions in 15 different countries (Table 2) and with nationalities from all inhabited continents have enrolled in the course.
To characterize the student experiences, we designed a questionnaire about the course and the NOR-R-AM project. Students who have enrolled in the course were invited to complete anonymous questionnaire about their experiences. Students were informed that the questionnaire was a part of a research project and that their participation was voluntary and anonymous. Students provided informed consent to participate in the project before beginning to fill out the questionnaire. The questionnaire was sent to all course participants from 2018–2023 for completion in February 2024. Overall, 27 of the 57 invitees (47 %) responded (5/15 from 2018, 4/13 from 2019, 11/15 from 2022, and 7/14 from 2023). In addition, four graduate students with significant NOR-R-AM involvement (i.e. active participation in at least three NOR-R-AM activities) were invited to provide discussion on the course based on their involvement and perspectives. These graduate students are co-investigators and co-authors in this project.
In addition to the pedagogical questionnaire designed for this contribution, UNIS conducts standardized course evaluations for every course. These provide useful information for the course responsible for optimizing the course from year to year. The recurring theme of these questionnaires for AG-x51 from 2019 to 2023 was that the field component was the highlight of the course.
6.1 Student experiences questionnaire
Figure 9 summarizes the quantitative student experiences. The response group covers all of the four years when the course was run (2018–2023), with 55 % of respondents being MSc students and 63 % of the respondents never having been above the Arctic Circle (Fig. 9a). There is, as usual, a mix of students choosing the course based on the learning objectives and the course being held at UNIS. Notably, the vast majority (82 %) of the respondents did not take a course on Arctic geology in the past. The student background was varied, including a mix of geologists, geophysicists, and economic geologists (Table 3).
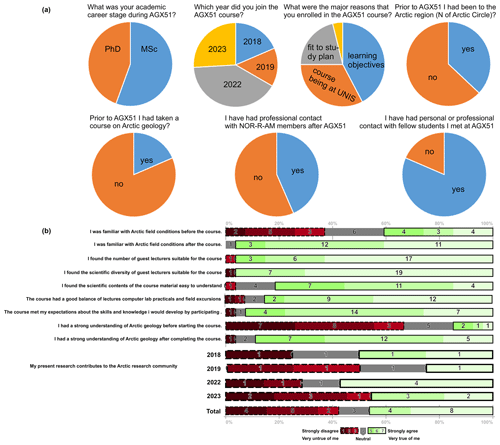
Figure 9Summary of the anonymous questionnaire circulated to past students of AGx51. Overall, 27 of 57 students responded. (a) Background of the respondents (in terms of career stage, year, and previous background) and post-course interaction with fellow students and the NOR-R-AM scientific team. (b) Responses to Likert-scale questions on the students' background and perspectives on various aspects of the course. For the statement “My present research contributes to the Arctic research community”, we have also investigated the difference per year. Likert-scale plots generated using Maurer (2013).
The course received largely positive feedback on the number and scientific diversity of guest lecturers and the balance between fieldwork, lectures, and seminars (Fig. 9b). The course contributed to improving the familiarity with Arctic field conditions of the respondents and providing an understanding of Arctic geology (Fig. 9b). Approximately half of the respondents still contribute to the Arctic research community.
Table 3 lists a selection of responses to the more open questions. Practical skills and knowledge learned during the course include both geoscientific concepts and also the active use of different software, and especially GPlates. Similarly, the biggest improvement experienced by the respondents was understanding not only geoscientific topics (with Arctic geology being the key improvement for many), but also data integration, software skills, and development of independent research projects. The teaching methods were well received, and the multi-disciplinary one-on-one supervision of research projects and active learning was mentioned by several students. The final student comments indicate suggestions for improvement (such as more fieldwork and focus on a single piece of software) and also demonstrate the non-academic impact of the course, for instance, on networking and the students' personal development and networking.
6.2 Collective and individual student experiences
We (Anna Sartell, Fenna Ammerlaan, Julian Janocha, and Rafael Horota) have each been involved in the AG-x51 course and the NOR-R-AM project at different stages of our research careers. We all participated in the course either as enrolled students during our MSc or PhD and/or have assisted as polar bear guards. These perspectives allow us to collectively reflect on the course and the opportunities that came from it.
The course was taught by members of the NOR-R-AM project, who are leading experts within their fields. This not only allowed us to learn about Arctic volcanism and tectonics from scientists with personal experience working in this region, but also gave us the opportunity to build professional relationships. This was made possible by utilizing the extensive time spent with our international peers and instructors during the course, including that during lectures, practical sessions, research projects, fieldwork, and social activities. This networking has led to many continuing personal development opportunities. To mention just a few of the opportunities, the course had profound implications on our career by collaborating with NOR-R-AM partners on our Master and PhD theses and even including some as official co-supervisors. Some of us were also able to join other NOR-R-AM courses, such as the geochronology workshop in Austin, Texas, or the field trip to Alaska. Moreover, NOR-R-AM was able to provide travel and analysis grants to accomplish the goals of the collaborations beyond those of the course. An overview of how the course functioned as a stepping stone for further involvement in the NOR-R-AM program and what impact this had on our individual career paths is illustrated by Fig. 10.
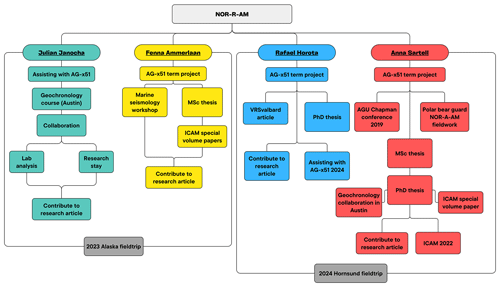
Figure 10Summary flowchart of the involvement of four selected students in the NOR-R-AM project, including the UNIS course and additional activities.
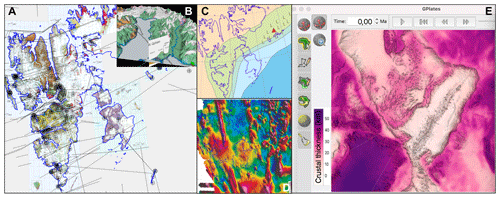
Figure 11Synthesis of the spatial and temporal elements provided in the supplementary material (Senger and Shephard, 2023). (a) Interactive geological map of Svalbard, also showing the location of published profiles (dashed lines; includes both seismic profiles and geological cross sections) and location of boreholes and selected published sedimentary logs from outcrops. (b) Zoom-in to a 3D view of Billefjorden where a digital terrain model was draped with a geological map and two profiles across the Billefjorden Fault Zone are co-visualized. (c) Published palaeogeographic map (Dallmann, 2015) from the Barremian (125 Ma) overlain with the extent of HALIP magmatism on Svalbard. (d) Published magnetic anomaly map (Dallmann, 2015). (e) An example of crustal thickness map at present day as illustrated in GPlates. The software also facilitates the digital visualization of tectonic plate reconstructions through geological time.
6.2.1 Anna Sartell
I took the AG-x51 course as a Master's student in summer 2019. The teaching comprised the lectures, practical sessions, field days, and term project. For me as a student, the course promoted active learning and a hands-on approach to the topics taught. The learning went beyond memorizing literature, and we rather learned how to use the knowledge we gained. The clear focus on practical learning throughout AG-x51 made this course very different to the rest of my education experience in a positive sense. The highlight of the course itself was the opportunity to work closely with one of the lecturers on our term project to expand further on what we had learned. Looking back, the biggest highlight has been the network that I gained from the course, which led to both my MSc and PhD theses being based on the topics of this course, HALIP, and including some of the lecturers as my supervisors.
6.2.2 Fenna Ammerlaan
In autumn 2022, I was a Master's student of the AG-x51 course. For me, the teaching approach in the different modules created a motivating environment as you recognized that you were being taught by experts in their respective fields. I believe this increased the effectiveness of the knowledge transfer even though the number of teaching staff involved sometimes resulted in some overlap between the lectures. My personal highlight was the fieldwork conducted. Integrating what can be abstract geological concepts with physical observations helps to fully understand the material taught in class. This course was unique for me due to the international environment, diversity in student and lecturer backgrounds, intensity of the course, and fieldwork. Combining this with the remoteness of Svalbard, it felt like being part of a small community rather than just attending a course. This community has since become an important part of my professional network and has been key in my academic career. It has resulted in a temporary research assistant job at UNIS, and I have since commenced a PhD working on North Atlantic geology in Norway.
6.2.3 Rafael Horota
I was involved with the AG-x51 course as both a student (in autumn 2022) and a polar bear guard (in spring 2023) during my PhD in higher education research. My involvement was motivated by the chance to gain firsthand experience from leading experts in Arctic volcanism and tectonics, and to apply cutting-edge technology like drone data collection in the context of geological Arctic field teaching. The teaching approach of the course, integrating lectures, practical sessions, research projects, fieldwork, and social activities, had positively impacted me as a student. Personal highlights were the possibility of collecting drone imagery, which was instrumental for my research, and the fact that it supported fieldwork. This experience, coupled with collaborations established through the course, has been invaluable for my professional and academic growth. Compared to other courses, the AG-x51 course stood out due to its hands-on approach and its fostering of international networks. The course's blend of traditional academic learning with innovative research methods and technology application provided a richer, more engaging learning environment than I had experienced anywhere else.
6.2.4 Julian Janocha
Whilst I was not a student in the AG-x51 course, I was employed as a student assistant in the summer of 2018 to work as a polar bear guard during field trips and to aid in the organization of social events. This was my first contact with the NOR-R-AM project. In autumn of 2019, I had the chance to participate in the geochronology short course organized at the University of Texas at Austin. This event was one of the most influential events in my research career. Learning about detrital zircon provenance and its applications inspired me to include it in my PhD project, which I started the following summer. This inspiration led to a 3-month long research stay at the University of Texas at Austin in the winter of 2022, during which I accomplished a detrital zircon provenance analysis for my PhD project and my participation in the Alaska field trip. The financial contributions by NOR-R-AM for both analysis and travel costs were essential for the success of this research stay. Overall NOR-R-AM has had a large influence on my professional career by offering courses, building a professional network, and providing financial support for collaborations.
7.1 Spatio-temporal perspective on Arctic evolution: teaching across country boundaries
Although our understanding of the geologic evolution of the Arctic is aided by geophysical surveys within the Arctic Ocean, we largely base our comprehension of this region on studies concerning the geology of the surrounding landmasses. Hence, within the Arctic, arguably more than anywhere else on Earth, there is a considerable need for cross-country research and teaching collaboration to get a more complete picture of the region's geologic evolution. This becomes even more apparent as we travel further back in time. For instance, our rather incomplete understanding of the opening of the Amerasian Basin in the Mesozoic requires correlation between tectonic and magmatic events and geologic units from the Canadian Arctic, Alaska, and northeastern Siberia (Shephard et al., 2013). Looking at earlier times, in order to understand the extent of the late Proterozoic/Early Paleozoic Timanian Orogen, we require a comprehensive review of sparse geologic evidence of this mountain-building event identified in locations such as Siberia, Scandinavia, North America, and numerous Arctic archipelagos (e.g. Svalbard, New Siberian Islands, and Severnaya Zemlya; e.g. Gee et al., 2006). The further we delve back in time, the more uncertain the reconstruction of the Arctic's geologic evolution becomes and the more a spatio-temporal perspective on Arctic evolution demands an interdisciplinary and collaborative effort that transcends national boundaries. The NOR-R-AM collaborative project has aimed to address this through providing numerous international educational opportunities (e.g. the course described in this contribution) in order to bring researchers and students from various Arctic countries together and to gain perspective on the geology of the Arctic regions from other countries.
In addition, the vast and remote landscapes of the Arctic, coupled with harsh climatic conditions, have limited the accessibility and comprehensive mapping of geological features and acquisition of field data. The scarcity of geoscience data highlights two needs within the Arctic community: (1) cross-country collaboration to generate a reliable database to store this patchwork of data and (2) availability of testable geodynamic models that take into account data that transcends international boundaries. Cross-country initiatives, such as the NOR-R-AM project, are necessary to bring together researchers from various nations (Table 2) to pool their expertise and resources. This collaborative approach recognizes that no single country possesses the entirety of the puzzle; instead, a mosaic of insights from different perspectives is required to construct a comprehensive narrative of the Arctic's geologic evolution.
7.2 Lessons learned: data, tools, software, and workflows
From the onset, we have designed the course with focus on active, hands-on learning at the expense of frontal lecture-based education. The culmination of this was the delivery of the student research projects where adequate (but not infinite) time is allocated to test a scientific hypothesis using the provided data and skill sets.
To make the course as authentic as possible, we teach and integrate a broad range of software and tools (Table 4). Obviously, there is insufficient time within a 6-week course period to go in depth regarding all of the relevant topics, and we take the approach to expose the entire class to as many tools and topics and possible and provide them with an active learning approach. For data mining purposes, the GPlates and SILLi programs are also linked to hands-on exercises for the entire class. For smaller student groups that use a specific software, sometimes only available under specific licenses, during their term projects, additional hands-on sessions are organized. In addition, we used a pre-course questionnaire to identify the strengths of individual students (for instance, significant experience in GPlates) who acted as additional tutors in the hands-on sessions to assist their peers.
Table 4Tools, software, and key datasets used in the course include a mix of free and open-software and proprietary software. All software programs or tools were accessible for all of the students (whether in the computer lab or via personal laptop) and were used for the individual student research projects.
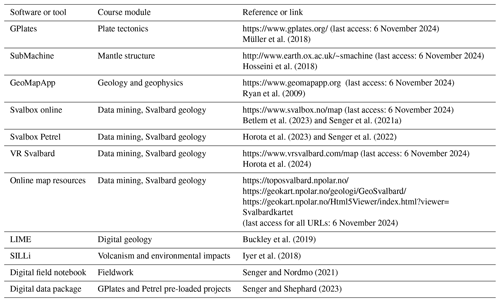
With such diverse software, we have devised pre-loaded projects in Petrel and GPlates software (Fig. 11), with the data packages available to anyone as part of the supplementary material (Senger and Shephard, 2023). Petrel is largely used to spatially integrate the surface (terrain models; bathymetry; and geological, topographical, and satellite maps) with the subsurface (borehole and geophysical data plus geomodels). The thematic dataset provided for the AGx51 builds on the ongoing Svalbox project. The key benefit of such direct integration is to spend less time on data loading and more time on the scientific benefits of data integration, for instance, in the AGx51 student projects. The integration of multi-physical data, for instance, geophysics with geology, also facilitates joint interpretation. Finally, the provision of curated (and regularly updated) databases that we do for the AGx51 course is even more important in the Arctic, where data are often fragmentary and sparsely acquired over a large area.
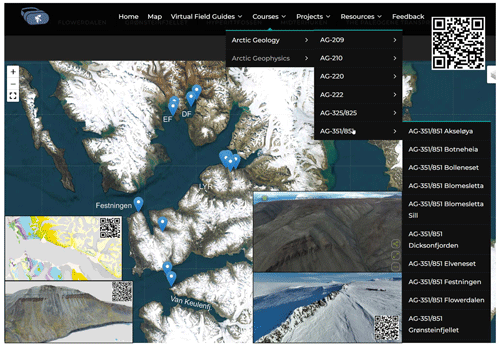
Figure 12Synthesis of data systematically acquired as part of the AG351/851 course and openly available on VR Svalbard (https://vrsvalbard.com/ag-351-851/, last access: 6 November 2024; access is via the main QR code). The geological inset map illustrates the photosphere and digital outcrop model coverage in Van Keulenfjorden where the Central Basin infill is well exposed. The interactive map at https://www.svalbox.no/map (last access: 6 November 2024) is accessible with the QR code. The low inset image illustrates a digital outcrop model of the 310 m high mountain Grønsteinfjellet visited during fieldwork in October 2022 (https://sketchfab.com/3d-models/grnsteinfjellet-7185d44b49d74a9daad35f438d52cf2a, last access: 6 November 2024). The Botneheia locality as visited in April 2023 provides an excellent exposure of a HALIP dyke. Photospheres taken in summer complement the winter snow-covered conditions and are freely available at https://vrsvalbard.com/botneheia/ (last access: 6 November 2024).
7.3 Transforming geoscience education through hands-on digital tools
The main motivation of exposing the students to such a wide range of software within a short time frame is to appreciate that geoscience is undergoing a digital transformation (Bouziat et al., 2020; Gunderson et al., 2020). McCaffrey et al. (2005) recognized that affordable digital technologies will revolutionize how field geology is conducted. Ruggedized tablets, as described from the Svalbard environment by Senger and Nordmo (2021) or lidar-equipped iPhone (Tavani et al., 2022) drone-based imagery, have led to the widespread adoption of digital outcrop modelling (Betlem et al., 2023). Geology has traditionally been an observation-focussed domain, with a focus on measuring nature at various scales, recording mostly qualitative data in the field. Through digitization, we bring quantitative and repeatable analyses into geology, for instance, through the active use of digital outcrop models or time-lapse digital tectonic plate reconstructions. Such efforts are necessary to not only gain a better understanding of Earth's evolution but also have the future added benefit of recruiting students to the geosciences and bridging the gap between geoscientists and data scientists.
The complex spatial–temporal tectono-magmatic evolution of Svalbard imposes logistical challenges to exemplify geological concepts in the field within the framework of the AG-x51 course. However, modern technology and digital tools provide innovative solutions to overcome these obstacles. Geospatial data and GIS are essential for creating detailed maps of the region, facilitating the teaching of concepts like tectonic evolution and volcanic processes. Indeed, 3-dimensional modelling and visualization tools allow for the creation of immersive models that aid in understanding complex geological structures by supporting 3-dimensional thinking. Remote sensing technologies, such as drones and satellites, provide real-time data and images, enabling students to change the observer's perspective when analysing large-scale geology. Digital workflows and analytical tools streamline data analysis, while online collaboration platforms enhance collective learning experiences. Virtual field trips offer a safe and accessible way for students to explore Svalbard's geological features, fostering a deeper understanding of the region's unique geology. In essence, the integration of these digital tools and workflows empowers students to engage in hands-on learning, regardless of the remote and challenging environment of Svalbard.
In the unforgiving Arctic field sites, such digital tools are almost a must to overcome the various challenges of field teaching at Svalbard (Senger et al., 2021b). However, lessons from the Arctic, be they technological, pedagogical, or both, can also be adapted at more temperate latitudes to improve accessibility (Whitmeyer et al., 2020; Atchison and Libarkin, 2013). We are also strong proponents that active and targeted digital geoscience tool usage in both education and outreach can significantly improve the diversity challenge faced by the geosciences (Hall et al., 2022).
7.4 Beyond the AG-x51 course: NOR-R-AM educational activities
In this contribution, we have focussed on the AG-x51 NOR-R-AM flagship course that also continues beyond the project period; however, Table 5 lists other educational and outreach activities undertaken as part of the NOR-R-AM project. The geographic and thematic diversity of these events testifies to the international and multi-disciplinary nature of the NOR-R-AM project.
In this contribution, we have outlined an international collaboration project, NOR-R-AM (Changes at the Top of the World through Volcanism and Plate Tectonics) and specifically focussed on a graduate course titled Arctic Tectonics and Volcanism that has been held annually at the University Centre in Svalbard since 2018. The presented article and the supplementary data package are intended to serve as a foundation for teaching Arctic geology elsewhere rather than at UNIS, albeit without the field component. We conclude the following:
-
Political and discipline boundaries must be set aside to comprehend the geological evolution of the Arctic.
-
Teaching Arctic geology requires provision of circum-Arctic data spanning both spatial (lateral and vertical) and temporal (i.e. geological evolution) scales.
-
The multi-disciplinary course titled Arctic Tectonics and Volcanism exposes the students to various tools and methods in order to decipher one particular aspect of Arctic geology through an individual research project.
-
Four NOR-R-AM students provided specific examples into how the course and the NOR-R-AM project impacted their respective careers, primarily through networking opportunities, grants, and supervision of research projects.
-
We provide three open-access datasets that may facilitate circum-Arctic geoscience teaching beyond UNIS.
The educational material associated with the course (i.e. Petrel and GPlates data packages) is freely available on the Zenodo repository (https://doi.org/10.5281/zenodo.10259590, Senger and Shephard, 2023). Other data are available on request by contacting the corresponding author.
KS: conceptualization, investigation, resources, data curation, writing (original draft), visualization, supervision, project administration, and funding acquisition. GS: investigation, resources, data curation, writing (original draft), visualization, supervision, and project administration. FA, OA, KI, JJ, MJ, MO, AnnS, and DS: writing (original draft). PA and MAVK: writing (review and editing) and supervision. RKH: writing (review and editing) and visualization. BC, VE, JIE, SAG, AM, and AndS: writing (review and editing). CG: writing (original draft), visualization, supervision, project administration, and funding acquisition.
The contact author has declared that none of the authors has any competing interests.
While the course focussed on a geopolitically and controversy-prone area, we do not foresee any ethical issues with this project as it pertains to students' experiences in a course. The questionnaire, which was both anonymous and voluntary, was developed in line with the Norwegian National Ethics Committee's Guidelines for Research Ethics in the Social Sciences and Humanities (which is the most relevant set of ethical guidelines for teaching-related research projects). Further, the project was reviewed by UNIS' internal ethical committee. No specific permission from a research ethics board is required for this kind of research in Norway and thus could not be sought. Four graduate students with significant NOR-R-AM involvement were invited as co-authors to this contribution based on their significant involvement in several activities.
Publisher’s note: Copernicus Publications remains neutral with regard to jurisdictional claims made in the text, published maps, institutional affiliations, or any other geographical representation in this paper. While Copernicus Publications makes every effort to include appropriate place names, the final responsibility lies with the authors.
We sincerely thank all the AGx51 students over the years for their contributions, including answering the questionnaire. UNIS logistics department supported all our field activities and undertook the necessary safety training. Schlumberger and Cegal generously provided an academic license for Petrel and the Blueback Toolbox, respectively. We thank the editor, Leslie Almberg; Aleksandra Smyrak-Sikora; and an anonymous reviewer for their comments which improved this paper.
The NOR-R-AM and NOR-R-AM2 projects were funded through the INTPART programme of the Research Council of Norway (grant nos. 261729 and 309477). We acknowledge support from the Research Council of Norway (RCN; project no. 223272) via the Centre for Earth Evolution and Dynamics (CEED; grant no. 332523), Centre for Planetary Habitability (PHAB; grant no. 326238), POLARIS – Evolution of the Arctic in Deep Time (grant no. 331679), Svalbox 2.0 – FAIR geoscientific data from Svalbard (grant no. 331679), and DYPOLE – Dynamics of polar confined basins (grant no. 325984). Additional funding was provided by the University of the Arctic (UArctic; project HALIPdat) and by UNIS.
This paper was edited by Leslie Almberg and reviewed by Aleksandra Smyrak-Sikora and one anonymous referee.
Abdelmalak, M. M., Gac, S., Faleide, J. I., Shephard, G. E., Tsikalas, F., Polteau, S., Zastrozhnov, D., and Torsvik, T. H.: Quantification and Restoration of the Pre-Drift Extension Across the NE Atlantic Conjugate Margins During the Mid-Permian-Early Cenozoic Multi-Rifting Phases, Tectonics, 42, e2022TC007386, https://doi.org/10.1029/2022TC007386, 2023.
Abdelmalak, M. M., Minakov, A., Faleide, J. I., and Drachev, S. S.: Lomonosov Ridge Composite Tectono-Sedimentary Element, Arctic Ocean, Geological Society, London, Memoirs, 57, M57-2022-2072, https://doi.org/10.1144/M57-2022-72, 2024.
Anell, I., Braathen, A., and Olaussen, S.: The Triassic-Early Jurassic of the northern Barents Shelf: a regional understanding of the Longyearbyen CO2 reservoir, Norw. J. Geol., 94, 83–98, 2014.
Anfinson, O. A., Leier, A. L., Embry, A. F., and Dewing, K.: Detrital zircon geochronology and provenance of the Neoproterozoic to Late Devonian Franklinian Basin, Canadian Arctic Islands, GSA Bulletin, 124, 415–430, https://doi.org/10.1130/b30503.1, 2012.
Anfinson, O. A., Odlum, M. L., Piepjohn, K., Poulaki, E. M., Shephard, G. E., Stockli, D. F., Levang, D., Jensen, M. A., and Pavlovskaia, E. A.: Provenance Analysis of the Andrée Land Basin and Implications for the Paleogeography of Svalbard in the Devonian, Tectonics, 41, e2021TC007103, https://doi.org/10.1029/2021TC007103, 2022.
Atchison, C. L. and Libarkin, J. C.: Fostering accessibility in geoscience training programs, Eos, Transactions American Geophysical Union, 94, 400-400, 2013.
Beranek, L. P., Gee, D. G., and Fisher, C. M.: Detrital zircon U-Pb-Hf isotope signatures of Old Red Sandstone strata constrain the Silurian to Devonian paleogeography, tectonics, and crustal evolution of the Svalbard Caledonides, GSA Bulletin, 132, 1987–2003, https://doi.org/10.1130/b35318.1, 2020.
Betlem, P., Rodes, N., Birchall, T., Dahlin, A., Smyrak-Sikora, A., and Senger, K.: The Svalbox Digital Model Database: a geoscientific window to the High Arctic, Geosphere, 19, 1640–1666, https://doi.org/10.1130/GES02606.1, 2023.
Blakey, R.: Paleotectonic and paleogeographic history of the Arctic region, Atl. Geol., 57, 7–39, https://doi.org/10.4138/atlgeol.2021.002, 2021.
Blischke, A., Brandsdóttir, B., Stoker, M. S., Gaina, C., Erlendsson, Ö., Tegner, C., Halldórsson, S. A., Helgadóttir, H. M., Gautason, B., Planke, S., Koppers, A. A. P., and Hopper, J. R.: Seismic Volcanostratigraphy: The Key to Resolving the Jan Mayen Microcontinent and Iceland Plateau Rift Evolution, Geochem. Geophy. Geosy., 23, e2021GC009948, https://doi.org/10.1029/2021GC009948, 2022.
Bouziat, A., Schmitz, J., Deschamps, R., and Labat, K.: Digital transformation and geoscience education: New tools to learn, new skills to grow, European Geologist European Geologist, 15, 15–19, 2020.
Boyden, J. A., Müller, R. D., Gurnis, M., Torsvik, T. H., Clark, J. A., Turner, M., Ivey-Law, H., Watson, R. J., and Cannon, J. S.: Next-generation plate-tectonic reconstructions using GPlates, in: Geoinformatics: Cyberinfrastructure for the Solid Earth Sciences, edited by: Keller, G. R. and Baru, C., Cambridge, Cambridge University Press, 95–113, https://doi.org/10.1017/CBO9780511976308.008, 2011.
Braathen, A., Bergh, S. G., and Maher, H. D., Jr: Application of a critical wedge taper model to the Tertiary transpressional fold-thrust belt on Spitsbergen, Svalbard, Geol. Soc. Am. Bull., 111, 1468–1485, 1999.
Brekke, H. and Banet, C.: The Law of the Seabed: Access, Uses, and Protection of Seabed Resources, in: Chapter 4 Setting Maritime Limits and Boundaries: Experiences from Norway, Brill | Nijhoff, 85–103, ISBN (e-book) 978-90-04-39156-7, 2020.
Brekke, T., Krajewski, K. P., and Hubred, J. H.: Organic geochemistry and petrography of thermally altered sections of the Middle Triassic Botneheia Formation on south-western Edgeøya, Svalbard, Norwegian Petroleum Directorate Bulletin, 11, 111–128, 2014.
Brustnitsyna, E., Ershova, V., Khudoley, A., Maslov, A., Andersen, T., Stockli, D., and Kristoffersen, M.: Age and provenance of the Precambrian Middle Timan clastic succession: Constraints from detrital zircon and rutile studies, Precambrian Res., 371, 106580, https://doi.org/10.1016/j.precamres.2022.106580, 2022.
Buckley, S. J., Ringdal, K., Naumann, N., Dolva, B., Kurz, T. H., Howell, J. A., and Dewez, T. J.: LIME: Software for 3-D visualization, interpretation, and communication of virtual geoscience models, Geosphere, 15, 222–235, doi.org/10.1130/GES02002.1, 2019.
Colpron, M. and Nelson, J. L.: A Palaeozoic Northwest Passage: incursion of Caledonian, Baltican and Siberian terranes into eastern Panthalassa, and the early evolution of the North American Cordillera, Geol. Soc. Lond. Spec. Publ., 318, 273–307, https://doi.org/10.1144/SP318.10, 2009.
Corfu, F., Polteau, S., Planke, S., Faleide, J. I., Svensen, H., Zayoncheck, A., and Stolbov, N.: U–Pb geochronology of Cretaceous magmatism on Svalbard and Franz Josef Land, Barents Sea large igneous province, Geol. Mag., 150, 1127–1135, 2013.
Dallmann, W.: Geoscience Atlas of Svalbard, Norsk Polarinstitutt Rapportserie, 148, 292, http://hdl.handle.net/11250/2580810 (last access: 7 December 2024), 2015.
Dallmann, W. K., Dypvik, H., Gjelberg, J. G., Harland, W. B., Johannessen, E. P., Keilen, H. B., Larssen, G. B., Lønøy, A., Midbøe, P. S., Mørk, A., Nagy, J., Nilsson, I., Nøttvedt, A., Olaussen, S., Pcelina, T. M., Steel, R. J., and Worsley, D.: Lithostratigraphic Lexicon of Svalbard: Review and recommendations for nomenclature use, edited by: Dallmann, W. K., Norsk Polarinstitutt, Tromsø, 318 pp., ISBN 8276661661, https://nhm2.uio.no/norges/litho/svalbard/refs.htm (last access: 7 December 2024), 1999.
Dodson, M. H.: Closure temperature in cooling geochronological and petrological systems, Contrib. Mineral. Petr., 40, 259–274, https://doi.org/10.1007/BF00373790, 1973.
Døssing, A., Gaina, C., and Brozena, J. M.: Building and breaking a large igneous province: An example from the High Arctic, Geophys. Res. Lett., 44, 6011–6019, https://doi.org/10.1002/2016GL072420, 2017.
Døssing, A., Gaina, C., Jackson, H. R., and Andersen, O. B.: Cretaceous ocean formation in the High Arctic, Earth Planet. Sc. Lett., 551, 116552, https://doi.org/10.1016/j.epsl.2020.116552, 2020.
Eidesen, P. and Hjelle, S. S.: How to make virtual field guides, and use them to bridge field-and classroom teaching, Authorea [preprint], https://doi.org/10.22541/au.168001737.79628030/v1, 2023.
Ershova, V., Anfinson, O., Prokopiev, A., Khudoley, A., Stockli, D., Faleide, J. I., Gaina, C., and Malyshev, N.: Detrital zircon (U-Th)/He ages from Paleozoic strata of the Severnaya Zemlya Archipelago: Deciphering multiple episodes of Paleozoic tectonic evolution within the Russian High Arctic, J. Geodynam., 119, 210–220, 2018.
Ershova, V., Prokopiev, A., Stockli, D., Kurapov, M., Kosteva, N., Rogov, M., Khudoley, A., and Petrov, E. O.: Provenance of the Mesozoic Succession of Franz Josef Land (North-Eastern Barents Sea): Paleogeographic and Tectonic Implications for the High Arctic, Tectonics, 41, e2022TC007348, https://doi.org/10.1029/2022TC007348, 2022.
Evenchick, C. A., Davis, W. J., Bédard, J. H., Hayward, N., and Friedman, R. M.: Evidence for protracted High Arctic large igneous province magmatism in the central Sverdrup Basin from stratigraphy, geochronology, and paleodepths of saucer-shaped sills, Geol. Soc. Am. Bull., 127, 1366–1390, https://doi.org/10.1130/b31190.1, 2015.
Ford, J. D., Pearce, T., Canosa, I. V., and Harper, S.: The rapidly changing Arctic and its societal implications, WIREs Clim. Change, 12, e735, https://doi.org/10.1002/wcc.735, 2021.
Gaina, C.: Arctic Continental Margins, Continental Rifted Margins 2: Case Examples, 133–148, 2022.
Gaina, C., Werner, S. C., Saltus, R., Maus, S., and the CAMP-GM GROUP: Chapter 3 Circum-Arctic mapping project: new magnetic and gravity anomaly maps of the Arctic, Geol. Soc. Lond. Mem., 35, 39–48, https://doi.org/10.1144/m35.3, 2011.
Gee, D.: Caledonides of Scandinavia, Greenland, and Svalbard, Reference Module in Earth Systems and Environmental Sciences, Elsevier, ISBN 9780124095489, https://doi.org/10.1016/B978-0-12-409548-9.09133-8, 2015.
Gee, D. G., Bogolepova, O. K., and Lorenz, H.: The Timanide, Caledonide and Uralide orogens in the Eurasian high Arctic, and relationships to the palaeo-continents Laurentia, Baltica and Siberia, Geol. Soc. Lond. Mem., 32, 507–520, https://doi.org/10.1144/GSL.MEM.2006.032.01.31, 2006.
Gilmullina, A., Klausen, T. G., Paterson, N. W., Suslova, A., and Eide, C. H.: Regional correlation and seismic stratigraphy of Triassic Strata in the Greater Barents Sea: Implications for sediment transport in Arctic basins, Basin Res., 33, 1546–1579, https://doi.org/10.1111/bre.12526, 2021.
Glørstad-Clark, E., Birkeland, E. P., Nystuen, J. P., Faleide, J. I., and Midtkandal, I.: Triassic platform-margin deltas in the western Barents Sea, Mar. Petrol. Geol., 28, 1294–1314, https://doi.org/10.1016/j.marpetgeo.2011.03.006, 2011.
Gold, A. U., Kirk, K., Morrison, D., Lynds, S., Sullivan, S. B., Grachev, A., and Persson, O.: Arctic Climate Connections Curriculum: A Model for Bringing Authentic Data Into the Classroom, Journal of Geoscience Education, 63, 185–197, https://doi.org/10.5408/14-030.1, 2015.
Gold, A. U., Pfirman, S., and Scowcroft, G. A.: The imperative for polar education, Journal of Geoscience Education, 69, 97–99, https://doi.org/10.1080/10899995.2021.1903242, 2021.
Grundvåg, S. A., Marin, D., Kairanov, B., Śliwińska, K. K., Nøhr-Hansen, H., Jelby, M. E., Escalona, A., and Olaussen, S.: The Lower Cretaceous succession of the northwestern Barents Shelf: Onshore and offshore correlations, Mar. Petrol. Geol., 86, 834–857, https://doi.org/10.1016/j.marpetgeo.2017.06.036, 2017.
Gunderson, K. L., Holmes, R. C., and Loisel, J.: Recent digital technology trends in geoscience teaching and practice, GSA Today, 30, 39–41, 2020.
Hall, C. A., Illingworth, S., Mohadjer, S., Roxy, M. K., Poku, C., Otu-Larbi, F., Reano, D., Freilich, M., Veisaga, M.-L., Valencia, M., and Morales, J.: GC Insights: Diversifying the geosciences in higher education: a manifesto for change, Geosci. Commun., 5, 275–280, https://doi.org/10.5194/gc-5-275-2022, 2022.
Harrison, J. C., St-Onge, M. R., Petrov, O., Strelnikov, S., Lopatin, B., Wilson, F., Tella, S., Paul, D., Lynds, T., Shokalsky, S., Hults, C., Bergman, S., Jepsen, H. F., and Solli, A.: Geological map of the Arctic, Geological Survey of Canada, Open File, 5816, https://doi.org/10.4095/287868, 2008.
Heininen, L., Everett, K., Padrtova, B., and Reissell, A.: Arctic Policies and Strategies – Analysis, Synthesis, and Trends, International Institute for Applied Systems Analysis, Laxenburg, Austria https://doi.org/10.22022/AFI/11-2019.16175, 2020.
Helland-Hansen, W. and Grundvåg, S.-A.: The Svalbard Eocene-Oligocene (?) Central Basin succession: Sedimentation patterns and controls, Basin Res., 33, 729–753, https://doi.org/10.1111/bre.12492, 2020.
Henriksen, E., Ryseth, A. E., Larssen, G. B., Heide, T., Rønning, K., Sollid, K., and Stoupakova, A. V.: Chapter 10 Tectonostratigraphy of the greater Barents Sea: implications for petroleum systems, in: Arctic Petroleum Geology, edited by: Spencer, A. M., Embry, A. F., Gautier, D. L., Stoupakova, A. V., and Sørensen, K., 1, The Geological Society, London, 163–195, 2011.
Hesselbo, S. P., Robinson, S. A., Surlyk, F., and Piasecki, S.: Terrestrial and marine extinction at the Triassic-Jurassic boundary synchronized with major carbon-cycle perturbation: A link to initiation of massive volcanism?, Geology, 30, 251–254, https://doi.org/10.1130/0091-7613(2002)030<0251:TAMEAT>2.0.CO;2, 2002.
Horota, R. K., Senger, K., Rodes, N., Betlem, P., Smyrak-Sikora, A., Jonassen, M. O., Kramer, D., and Braathen, A.: West Spitsbergen fold and thrust belt: A digital educational data package for teaching structural geology, J. Struct. Geol., 167, 104781, https://doi.org/10.1016/j.jsg.2022.104781, 2023.
Horota, R. K., Senger, K., Smyrak-Sikora, A., Furze, M., Retelle, M., Kloet, M. A. V., and Jonassen, M. O.: VR Svalbard – a photosphere-based atlas of a high Arctic geo-landscape, First Break, 42, 35–42, https://doi.org/10.3997/1365-2397.fb2024029, 2024.
Hosseini, K., Matthews, K. J., Sigloch, K., Shephard, G. E., Domeier, M., and Tsekhmistrenko, M.: SubMachine: Web-Based Tools for Exploring Seismic Tomography and Other Models of Earth's Deep Interior, Geochem. Geophy. Geosy., 19, 1464–1483, https://doi.org/10.1029/2018GC007431, 2018.
Iyer, K., Svensen, H., and Schmid, D. W.: SILLi 1.0: a 1-D numerical tool quantifying the thermal effects of sill intrusions, Geosci. Model Dev., 11, 43–60, https://doi.org/10.5194/gmd-11-43-2018, 2018.
Jakobsson, M., Mayer, L., Coakley, B., Dowdeswell, J. A., Forbes, S., Fridman, B., Hodnesdal, H., Noormets, R., Pedersen, R., Rebesco, M., Schenke, H. W., Zarayskaya, Y., Accettella, D., Armstrong, A., Anderson, R. M., Bienhoff, P., Camerlenghi, A., Church, I., Edwards, M., Gardner, J. V., Hall, J. K., Hell, B., Hestvik, O., Kristoffersen, Y., Marcussen, C., Mohammad, R., Mosher, D., Nghiem, S. V., Pedrosa, M. T., Travaglini, P. G., and Weatherall, P.: The International Bathymetric Chart of the Arctic Ocean (IBCAO) Version 3.0, Geophys. Res. Lett., 39, L12609, https://doi.org/10.1029/2012GL052219, 2012.
Jones, M. T., Augland, L. E., Shephard, G. E., Burgess, S. D., Eliassen, G. T., Jochmann, M. M., Friis, B., Jerram, D. A., Planke, S., and Svensen, H. H.: Constraining shifts in North Atlantic plate motions during the Palaeocene by U-Pb dating of Svalbard tephra layers, Sci. Rep., 7, 6822, https://doi.org/10.1038/s41598-017-06170-7, 2017.
Khudoley, A. K., Sobolev, N. N., Petrov, E. O., Ershova, V. B., Makariev, A. A., Makarieva, E. V., Gaina, C., and Sobolev, P. O.: A reconnaissance provenance study of Triassic–Jurassic clastic rocks of the Russian Barents Sea, GFF, 141, 263–271, https://doi.org/10.1080/11035897.2019.1621372, 2019.
Klausen, T. G., Nyberg, B., and Helland-Hansen, W.: The largest delta plain in Earth's history, Geology, 47, 470–474, https://doi.org/10.1130/G45507.1, 2019.
Kristoffersen, Y.: Chapter 45 Geophysical exploration of the Arctic Ocean: the physical environment, survey techniques and brief summary of knowledge, Geol. Soc. Lond. Mem., 35, 685–702, https://doi.org/10.1144/m35.45, 2011.
Kristoffersen, Y., Nilsen, E. H., and Hall, J. K.: The High Arctic Large Igneous Province: first seismic-stratigraphic evidence for multiple Mesozoic volcanic pulses on the Lomonosov Ridge, central Arctic Ocean, J. Geol. Soc., 180, jgs2022-2153, https://doi.org/10.1144/jgs2022-153, 2023.
Krumpen, T., von Albedyll, L., Goessling, H. F., Hendricks, S., Juhls, B., Spreen, G., Willmes, S., Belter, H. J., Dethloff, K., Haas, C., Kaleschke, L., Katlein, C., Tian-Kunze, X., Ricker, R., Rostosky, P., Rückert, J., Singha, S., and Sokolova, J.: MOSAiC drift expedition from October 2019 to July 2020: sea ice conditions from space and comparison with previous years, The Cryosphere, 15, 3897–3920, https://doi.org/10.5194/tc-15-3897-2021, 2021.
Kurapov, M., Ershova, V., Khudoley, A., Luchitskaya, M., Stockli, D., Makariev, A., Makarieva, E., and Vishnevskaya, I.: Latest Permian–Triassic magmatism of the Taimyr Peninsula: New evidence for a connection to the Siberian Traps large igneous province, Geosphere, 17, 2062–2077, https://doi.org/10.1130/ges02421.1, 2021.
Lebedeva-Ivanova, N., Gaina, C., Minakov, A., and Kashubin, S.: ArcCRUST: Arctic Crustal Thickness From 3-D Gravity Inversion, Geochem. Geophy. Geosy., 20, 3225–3247, https://doi.org/10.1029/2018GC008098, 2019.
Lord, G., Janocha, J., Rodes, N., and Betlem, P.: Svalbox-DOM_2020-0015_Lagmannstoppen-East, Zenodo [data set], https://doi.org/10.5281/zenodo.5700918, 2021.
Lundschien, B. A., Høy, T., and Mørk, A.: Triassic hydrocarbon potential in the Northern Barents Sea; integrating Svalbard and stratigraphic core data, Norwegian Petroleum Directorate Bulletin, 11, 3–20, 2014.
Malm, R. H.: Developing an Arctic Geology course: exploring the role of fieldwork in a challenging learning space, Uniped, 44, 178–189, https://doi.org/10.18261/issn.1893-8981-2021-03-04, 2021.
Maurer, M. E.: likertplot.com – Plot Likert Scales”, http://likertplot.com/ (last access: 7 December 2024), 2013.
McCaffrey, K., Jones, R., Holdsworth, R., Wilson, R., Clegg, P., Imber, J., Holliman, N., and Trinks, I.: Unlocking the spatial dimension: digital technologies and the future of geoscience fieldwork, J. Geol. Soc., 162, 927–938, 2005.
Midtkandal, I., Faleide, J. I., Faleide, T. S., Serck, C. S., Planke, S., Corseri, R., Dimitriou, M., and Nystuen, J. P.: Lower Cretaceous Barents Sea strata: epicontinental basin configuration, timing, correlation and depositional dynamics, Geol. Mag., 157, 458–476, https://doi.org/10.1017/S0016756819000918, 2019.
Müller, R. D., Cannon, J., Qin, X., Watson, R. J., Gurnis, M., Williams, S., Pfaffelmoser, T., Seton, M., Russell, S. H. J., and Zahirovic, S.: GPlates: Building a Virtual Earth Through Deep Time, Geochem. Geophy. Geosy., 19, 2243–2261, https://doi.org/10.1029/2018GC007584, 2018.
Nikishin, A. M., Gaina, C., Petrov, E. I., Malyshev, N. A., and Freiman, S. I.: Eurasia Basin and Gakkel Ridge, Arctic Ocean: Crustal asymmetry, ultra-slow spreading and continental rifting revealed by new seismic data, Tectonophysics, 746, 64–82, https://doi.org/10.1016/j.tecto.2017.09.006, 2018.
Nordregio: Indigenous population in the Arctic, https://nordregio.org/maps/indigenous-population-in-the-arctic/ (last access: 7 December 2024), 2019.
Olaussen, S., Grundvåg, S.-A., Senger, K., Anell, I., Betlem, P., Birchall, T., Braathen, A., Dallmann, W., Jochmann, M., Johannessen, E. P., Lord, G., Mørk, A., Osmundsen, P. T., Smyrak-Sikora, A., and Stemmerik, L.: Svalbard Composite Tectono-Sedimentary Element, Barents Sea, Geol. Soc. Lond. Mem., 57, M57-2021-2036, https://doi.org/10.1144/M57-2021-36, 2024.
Petrov, O. V., Pubellier, M., Shokalsky, S. P., Morozov, A. F., Kazmin, Y. B., Kashubin, S. N., Vernikovsky, V. A., Smelror, M., Brekke, H., Kaminsky, V. D., and Pospelov, I. I.: New Tectonic Map of the Arctic, in: Tectonics of the Arctic, edited by: Petrov, O. V., and Smelror, M., Springer International Publishing, Cham, 1–27, https://doi.org/10.1130/2018.2541(08), 2021.
Piepjohn, K., von Gosen, W., and Tessensohn, F.: The Eurekan deformation in the Arctic: an outline, J. Geol. Soc., 173, 1007–1024, 2016.
Proelss, A.: Governing the Arctic Ocean, Nat. Geosci., 2, 310–313, https://doi.org/10.1038/ngeo510, 2009.
Prokopiev, A. V., Ershova, V. B., Anfinson, O., Stockli, D., Powell, J., Khudoley, A. K., Vasiliev, D. A., Sobolev, N. N., and Petrov, E. O.: Tectonics of the New Siberian Islands archipelago: Structural styles and low-temperature thermochronology, J. Geodynam., 121, 155–184, https://doi.org/10.1016/j.jog.2018.09.001, 2018.
Prokopiev, A. V., Ershova, V. B., and Stockli, D. F.: Provenance of the Devonian–Carboniferous clastics of the southern part of the Prikolyma terrane (Verkhoyansk–Kolyma orogen) based on U–Pb dating of detrital zircons, GFF, 141, 272–278, https://doi.org/10.1080/11035897.2019.1621373, 2019.
Ritsema, J., Deuss, A., van Heijst, H. J., and Woodhouse, J. H.: S40RTS: a degree-40 shear-velocity model for the mantle from new Rayleigh wave dispersion, teleseismic traveltime and normal-mode splitting function measurements, Geophys. J. Int., 184, 1223–1236, https://doi.org/10.1111/j.1365-246X.2010.04884.x, 2011.
Rogov, M. A., Ershova, V. B., Shchepetova, E. V., Zakharov, V. A., Pokrovsky, B. G., and Khudoley, A. K.: Earliest Cretaceous (late Berriasian) glendonites from Northeast Siberia revise the timing of initiation of transient Early Cretaceous cooling in the high latitudes, Cretaceous Res., 71, 102–112, 2017.
Rogov, M. M., Ershova, V., Gaina, C., Vereshchagin, O., Vasileva, K., Mikhailova, K., and Krylov, A.: Glendonites throughout the Phanerozoic, Earth-Sci. Rev., 241, 104430, https://doi.org/10.1016/j.earscirev.2023.104430, 2023a.
Rogov, M. A., Panchenko, I. V., Augland, L. E., Ershova, V. B., and Yashunsky, V. Y.: The first CA-ID-TIMS U-Pb dating of the Tithonian/Berriasian boundary beds in a Boreal succession, Gondwana Res., 118, 165–173, https://doi.org/10.1016/j.gr.2023.02.010, 2023b.
Ryan, W. B. F., Carbotte, S. M., Coplan, J. O., O'Hara, S., Melkonian, A., Arko, R., Weissel, R. A., Ferrini, V., Goodwillie, A., Nitsche, F., Bonczkowski, J., and Zemsky, R.: Global multi‐resolution topography synthesis, Geochem. Geophy. Geosy., 10, Q03014, https://doi.org/10.1029/2008GC002332, 2009.
Saltus, R. W., Miller, E. L., Gaina, C., and Brown, P. J.: Chapter 4 Regional magnetic domains of the Circum-Arctic: a framework for geodynamic interpretation, Geol. Soc. Lond. Mem., 35, 49–60, https://doi.org/10.1144/m35.4, 2011.
Schaeffer, A. J. and Lebedev, S.: Global shear speed structure of the upper mantle and transition zone, Geophys. J. Int., 194, 417–449, https://doi.org/10.1093/gji/ggt095, 2013.
Schneider, D. A., Faehnrich, K., Majka, J., Manecki, M., Piepjohn, K., Strauss, J. V., Reinhardt, L., and McClelland, W. C.: 40Ar/39Ar geochronologic evidence of Eurekan deformation within the West Spitsbergen Fold and Thrust Belt, in: Circum-Arctic Structural Events: Tectonic Evolution of the Arctic Margins and Trans-Arctic Links with Adjacent Orogens, Geol. Soc. Am., https://doi.org/10.1130/2018.2541(08), 2019.
Senger, K. and Galland, O.: Stratigraphic and Spatial extent of HALIP Magmatism in central Spitsbergen, Geochem. Geoph. Geosy., 23, e2021GC010300, https://doi.org/10.1029/2021GC010300, 2022.
Senger, K. and Shephard, G.: Arctic Tectonics and Volcanism: a multi-scale, multidisciplinary educational approach (Petrel and GPlates data package), Zenodo [data set], https://doi.org/10.5281/zenodo.10259590, 2023.
Senger, K. and Nordmo, I.: Using digital field notebooks in geoscientific learning in polar environments, Journal of Geoscience Education, 69, 166–177, https://doi.org/10.1080/10899995.2020.1725407, 2021.
Senger, K., Planke, S., Polteau, S., Ogata, K., and Svensen, H.: Sill emplacement and contact metamorphism in a siliciclastic reservoir on Svalbard, Arctic Norway, Norw. J. Geol., 94, 155–169, 2014a.
Senger, K., Tveranger, J., Ogata, K., Braathen, A., and Planke, S.: Late Mesozoic magmatism in Svalbard: A review, Earth-Sci. Rev., 139, 123–144, 2014b.
Senger, K., Betlem, P., Birchall, T., Buckley, S. J., Coakley, B., Eide, C. H., Flaig, P. P., Forien, M., Galland, O., Gonzaga Jr., L., Jensen, M., Kurz, T., Lecomte, I., Mair, K., Malm, R., Mulrooney, M., Naumann, N., Nordmo, I., Nolde, N., Ogata, K., Rabbel, O., Schaaf, N. W., and Smyrak-Sikora, A.: Using digital outcrops to make the high Arctic more accessible through the Svalbox database, Journal of Geoscience Education, 69, 123–137, https://doi.org/10.1080/10899995.2020.1813865, 2021a.
Senger, K., Betlem, P., Grundvåg, S.-A., Horota, R. K., Buckley, S. J., Smyrak-Sikora, A., Jochmann, M. M., Birchall, T., Janocha, J., Ogata, K., Kuckero, L., Johannessen, R. M., Lecomte, I., Cohen, S. M., and Olaussen, S.: Teaching with digital geology in the high Arctic: opportunities and challenges, Geosci. Commun., 4, 399–420, https://doi.org/10.5194/gc-4-399-2021, 2021b.
Senger, K., Betlem, P., Birchall, T., Gonzaga Jr., L., Grundvåg, S.-A., Horota, R. K., Laake, A., Kuckero, L., Mørk, A., Planke, S., Rodes, N., and Smyrak-Sikora, A.: Digitising Svalbard's Geology: the Festningen Digital Outcrop Model, First Break, 40, 47–55, https://doi.org/10.3997/1365-2397.fb2022021, 2022.
Senger, K., Kulhanek, D., Jones, M. T., Smyrak-Sikora, A., Planke, S., Zuchuat, V., Foster, W. J., Grundvåg, S.-A., Lorenz, H., Ruhl, M., Sliwinska, K. K., Vickers, M. L., and Xu, W.: Deep-time Arctic climate archives: high-resolution coring of Svalbard's sedimentary record – SVALCLIME, a workshop report, Sci. Dril., 32, 113–135, https://doi.org/10.5194/sd-32-113-2023, 2023.
Serreze, M. C. and Barry, R. G.: Processes and impacts of Arctic amplification: A research synthesis, Global Planet. Change, 77, 85–96, https://doi.org/10.1016/j.gloplacha.2011.03.004, 2011.
Shephard, G. E., Müller, R. D., and Seton, M.: The tectonic evolution of the Arctic since Pangea breakup: Integrating constraints from surface geology and geophysics with mantle structure, Earth-Sci. Rev., 124, 148–183, 2013.
Smyrak-Sikora, A., Johannessen, E. P., Olaussen, S., Sandal, G., and Braathen, A.: Sedimentary architecture during Carboniferous rift initiation–the arid Billefjorden Trough, Svalbard, J. Geol. Soc., 176, 225–252, 2019.
Straume, E. O., Gaina, C., Medvedev, S., and Nisancioglu, K. H.: Global Cenozoic Paleobathymetry with a focus on the Northern Hemisphere Oceanic Gateways, Gondwana Res., 86, 126–143, https://doi.org/10.1016/j.gr.2020.05.011, 2020.
Straume, E. O., Nummelin, A., Gaina, C., and Nisancioglu, K. H.: Climate transition at the Eocene–Oligocene influenced by bathymetric changes to the Atlantic–Arctic oceanic gateways, P. Natl. Acad. Sci. USA, 119, e2115346119, https://doi.org/10.1073/pnas.2115346119, 2022.
Struijk, E. L. M., Tesauro, M., Lebedeva-Ivanova, N. N., Gaina, C., Beekman, F., and Cloetingh, S. A. P. L.: The Arctic lithosphere: Thermo-mechanical structure and effective elastic thickness, Global Planet. Change, 171, 2–17, https://doi.org/10.1016/j.gloplacha.2018.07.014, 2018.
Svensen, H., Planke, S., Malthe-Sorenssen, A., Jamtveit, B., Myklebust, R., Rasmussen Eidem, T., and Rey, S. S.: Release of methane from a volcanic basin as a mechanism for initial Eocene global warming, Nature, 429, 542–545, 2004.
Tavani, S., Billi, A., Corradetti, A., Mercuri, M., Bosman, A., Cuffaro, M., Seers, T., and Carminati, E.: Smartphone assisted fieldwork: Towards the digital transition of geoscience fieldwork using LiDAR-equipped iPhones, Earth-Sci. Rev., 227, 103969, https://doi.org/10.1016/j.earscirev.2022.103969, 2022.
Topp-Jørgensen, E., Holste, S., Duveau, S. D., Ibáñez, M. M., Vural, D., Duncan, R., Gremion, G., Hancock, S., Rosenbaum, P., Burmenko, T., and Thomas, F. A.: INTERACT Reducing CO2 Emissions in Arctic Science, INTERACT, https://eu-interact.org/app/uploads/2022/10/INTERACTCo2-reduction-guidebook_FINAL-WORK_DPS.pdf (last access: 7 December 2024), 2022.
Vasileva, K., Zaretskaya, N., Ershova, V., Rogov, M., Stockli, L. D., Stockli, D., Khaitov, V., Maximov, F., Chernyshova, I., Soloshenko, N., Frishman, N., Panikorovsky, T., and Vereshchagin, O.: New model for seasonal ikaite precipitation: Evidence from White Sea glendonites, Mar. Geol., 449, 106820, https://doi.org/10.1016/j.margeo.2022.106820, 2022.
Whitmeyer, S. J., Atchison, C., and Collins, T. D.: Using Mobile Technologies to Enhance Accessibility and Inclusion in Field-Based Learning, GSA Today, 30, 4–10, https://doi.org/10.1130/GSATG462A.1, 2020.
Wignall, P. B.: Large igneous provinces and mass extinctions, Earth-Sci. Rev., 53, 1–33, https://doi.org/10.1016/s0012-8252(00)00037-4, 2001.
- Abstract
- Introduction
- NOR-R-AM and NOR-R-AM2 projects
- The AG-x51 course: motivation, establishment, incremental optimization, and limitations
- The AG-x51 course: modules
- The AG-x51 course: assessment
- Student perspectives
- Discussion
- Conclusions
- Data availability
- Author contributions
- Competing interests
- Ethical statement
- Disclaimer
- Acknowledgements
- Financial support
- Review statement
- References
- Abstract
- Introduction
- NOR-R-AM and NOR-R-AM2 projects
- The AG-x51 course: motivation, establishment, incremental optimization, and limitations
- The AG-x51 course: modules
- The AG-x51 course: assessment
- Student perspectives
- Discussion
- Conclusions
- Data availability
- Author contributions
- Competing interests
- Ethical statement
- Disclaimer
- Acknowledgements
- Financial support
- Review statement
- References